29.09.2016
-
HOW ROSETTA GETS PASSIVATED
The post below was sent in by Rosetta Spacecraft Operations Engineer Tiago Francisco, in response to our question: how does Rosetta get shut down upon comet landing?
ITU regulations require us to permanently switch off the craft's radio transmitter at end of mission. Since Rosetta wasn't designed to have its transmitter permanently off, we had to change the on-board software by patching it.
Basically, we expect the spacecraft to touch the surface, causing an attitude perturbation, and we expect this to trigger a safe mode.
Specifically, with the software change we created, once the spacecraft hits the surface of the comet, it will trigger an ‘FDIR’ response (Failure Detection, Isolation and Recovery – basically, the core on-board software that reacts when any monitored parameter goes out of limits), which will lead to a ‘safe mode’.
Upon completion of the safe-mode sequence, the spacecraft will be ‘passivated’ by using a specific branch nominally used for ground testing only. In other words, the craft will be placed into a passive, non-reactive mode that was initially designed only for ground testing prior to launch.
This means that all of the attitude and orbit control units will be off, as well as the transmitter.
As of yesterday, the software patch has been installed on board, but is not active. The first step to activate this response is being done today. Thereafter, the spacecraft will be passivated only if 15 reboots occur in a row (which is very unlikely). Three hours before the expected impact on 30 September, what we internally call the ‘point of no return’, we will fully activate the passivation instructions. Any safe mode after that point will passivate the spacecraft.
Upon landing, an FDIR trigger (likely caused by excess rates or off-pointing of the spacecraft) will cause a safe mode and hence the passivation.
---
LIVING WITH A COMET: AN OSIRIS TEAM PERSPECTIVE
OSIRIS, Rosetta’s Optical, Spectroscopic and Infrared Remote Imaging System, has been our all-seeing eye on Comet 67P/Churyumov-Gerasimenko, capturing nearly 68,000 high-resolution images of its nucleus and coma from all angles for 924 days. Here the OSIRIS team share some insights beyond the beauty of the images their camera returns. With inputs from Holger Sierks, OSIRIS principal investigator.
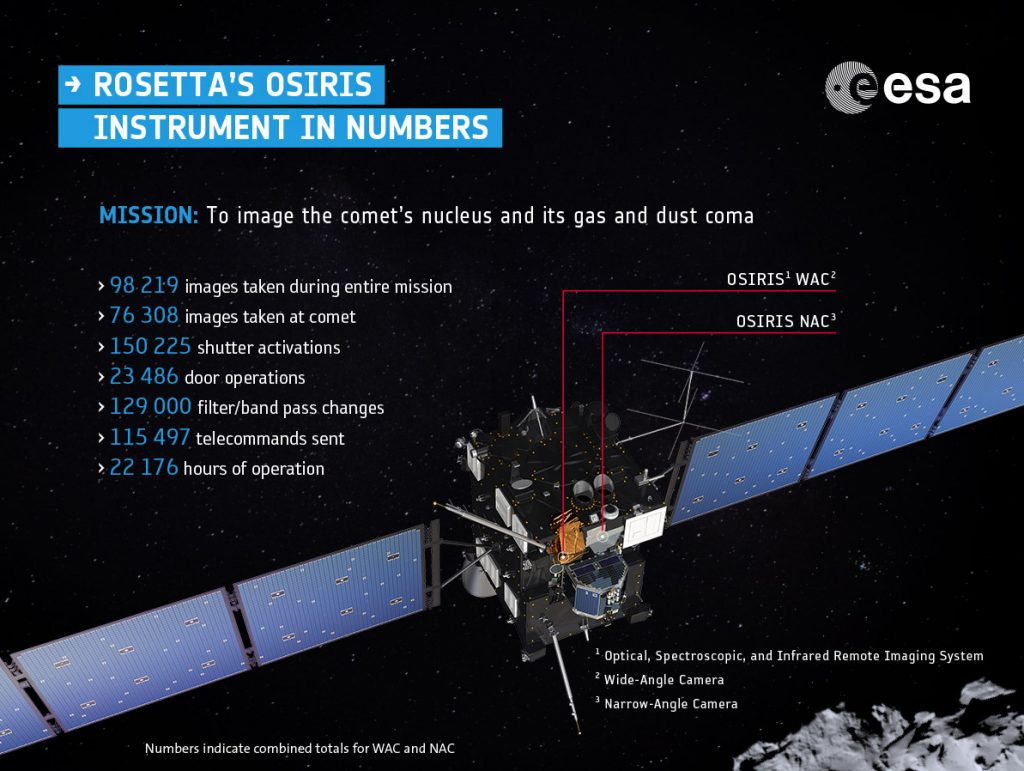
Numbers indicate combined totals for WAC and NAC. The breakdown is as follows: Shutter activations: NAC: 39,917; WAC: 110,308; Door operations: NAC: 11,560; WAC: 11,926; Filter/band pass changes: NAC: 72,727; WAC: 56,273. Of the 76,308 images taken at the comet, 67,905 are science images and the remainder technical images for calibration and testing.
OSIRIS has always had a big team: there are 97 team members today, and more than 300 people were involved – including industry partners – at the time the camera system was built. The instrument itself comprises two cameras – a wide- and narrow-angle camera, three electronics boxes, eight harnesses, and 22 subsystems, which were provided by nine European research institutes, plus industry, and with the support of six space agencies.
This massive endeavour ultimately resulted in a grand total of 98,219 images taken throughout the entire mission, including beautiful images of the Earth and Moon during Earth flybys and one at Mars, and never-before seen views of asteroids Steins and Lutetia, even before starting the main mission at Comet 67P/C-G.
OSIRIS set sights on Comet 67P/C-G for the first time since waking up from its 31-deep space hibernation at the end of March 2014 (it had already imaged the comet in March 2011, just a few months before hibernation), and since then has taken 76,308 images. Of those, 67,905 are science images capturing the comet nucleus and its gas and dust coma, with the remainder technical images for calibration and testing. These were executed thanks to 115,497 commands transmitted to the spacecraft.
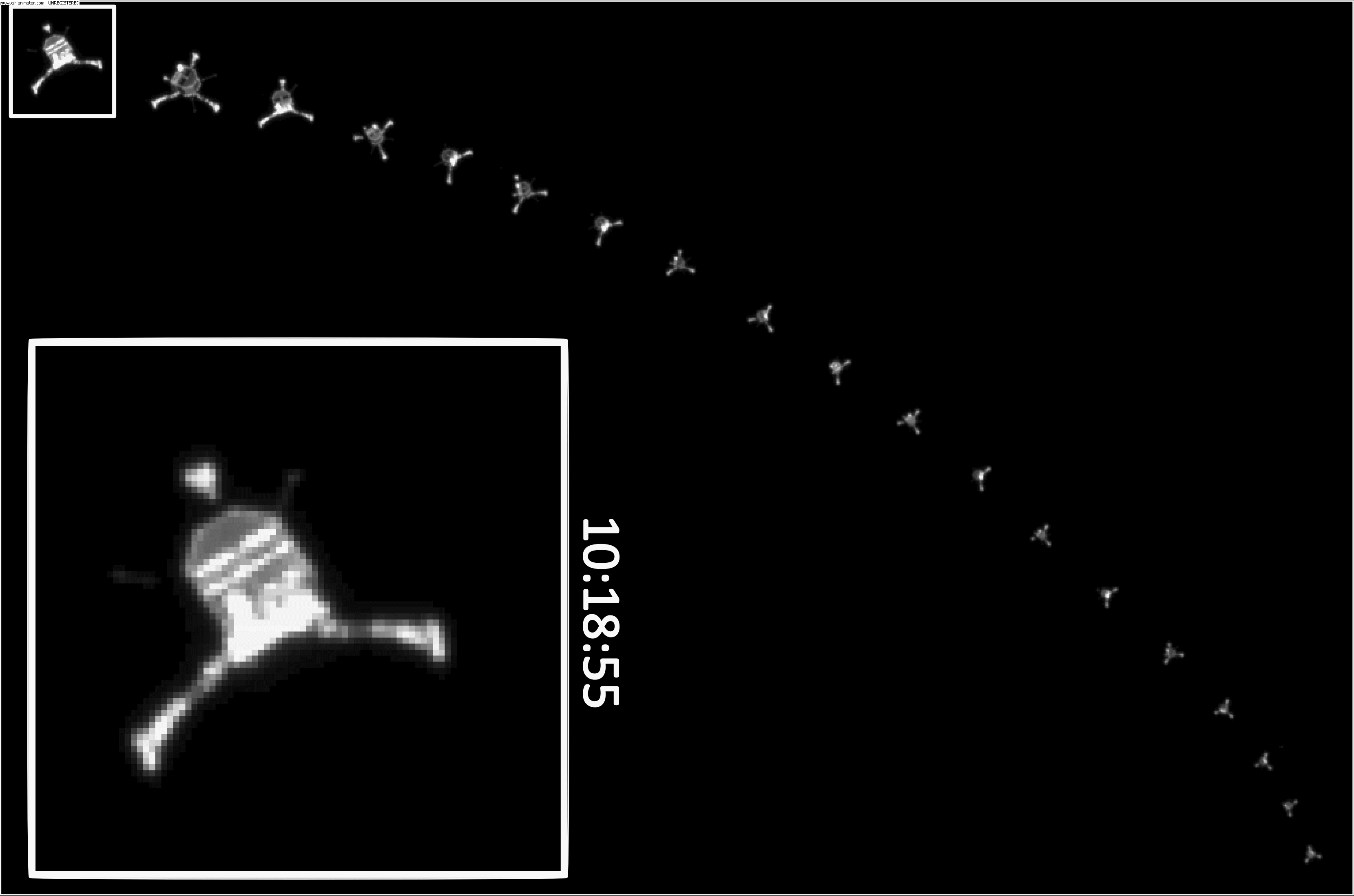
Philae falls away from Rosetta – as seen by the orbiter's OSIRIS camera. Credits: ESA/Rosetta/MPS for OSIRIS Team MPS/UPD/LAM/IAA/SSO/INTA/UPM/DASP/IDA
In amongst those tens of thousands of comet images are a handful that captured the public’s attention for another reason: Philae. They also hold a special place with the OSIRIS team:
“I was most touched by the ‘free-floating’ Philae after separation, then later when we saw Philae floating above surface, and finally, earlier this month, Philae in its final resting place. It is beyond science…” says Holger.
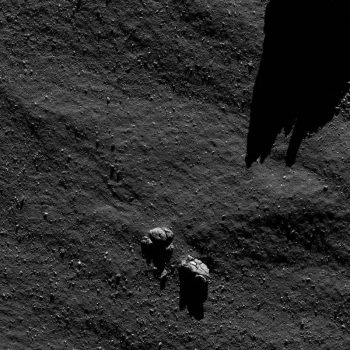
This image was taken on 23 September by the OSIRIS narrow-angle camera. Click for details via OSIRIS image of the day website. Credits: ESA/Rosetta/MPS for OSIRIS Team MPS/UPD/LAM/IAA/SSO/INTA/UPM/DASP/IDA
Also beyond science, but this time for more practical reasons, is the instrument’s housekeeping reporting: the coldest temperature recorded by OSIRIS was -124ºC – this was the measured NAC CCD temperature. By contrast, the highest measured temperature was +43ºC, at the WAC CRB (CCD readout board) power converter.
And, as with all of Rosetta’s instruments, there were plenty of bumps in the road: some 232 ‘non-conformance’ reports were generated in ground testing and during the mission.
Holger tells us that on the shelves at his home institute (the Max-Planck Institute for Solar System Research) alone, documentation concerning manuals, manufacturing and design documents, test reports, minutes of meetings and so on, span a length of about 40 metres!
Not included in that number is the impressive haul of scientific papers based on OSIRIS data: 83 completed so far, with another 31 currently in preparation.
We’re certainly looking forward to seeing what discoveries are still to come, and of course all eyes will be on the images that this incredible camera return during Rosetta’s final descent to the surface of the comet, tomorrow.
This post is part of a series that looks behind the scenes of the instrument teams to find out what it was really like "living with a comet" for two years.
---
SCIENCE HIGHLIGHTS BRIEFING STARTING SOON
The Rosetta science highlight briefing at the European Space Operations Centre (ESOC) in Darmstadt, Germany, will start shortly.
Tune in from 14:30 14:45 to the livestream viewer at rosetta.esa.int or via https://livestream.com/ESA/rosettagrandfinale or ESA's Facebook page to follow dedicated talks celebrating the scientific highlights of the mission.
Programme overview
- Matt Taylor (ESA’s Rosetta Project Scientist): Introduction
- Mohamed El-Maarry (OSIRIS team, University of Bern): Landscapes of Chury
- Valerie Ciarletti (CONSERT team, Universités Paris-Saclay): Getting the ground truth about the nucleus
- Thurid Mannel (MIDAS team, University of Graz): Dust under the microscope
- Jean-Baptiste Vincent (OSIRIS team, Max-Planck Institute for Solar Physics, Göttingen): Cometary activity and fireworks
- Andre Bieler (ROSINA team, University of Bern/University of Michigan): Comet activity variation and evolution
- Charlotte Goetz (RPC team, Institute for Extra-terrestrial Physics, TU Braunschweig): The singing comet
- Cecila Tubiana (OSIRIS team, Max-Planck Institute for Solar Physics, Göttingen): Rosetta’s link to Earth
- Kathrin Altwegg (ROSINA team, University of Bern): The cometary zoo
- Björn Davidsson (Asteroids, Comets and Satellites Group, JPL): Formation of our Solar System
- Matt Taylor: Final comments and close
---
BENEATH THE SURFACE OF COMET 67P
While scientists and the public alike have been astounded by the unexpected shape of Comet 67P/ Churyumov-Gerasimenko’s nucleus, what lies beneath the surface is just as important scientifically.
Comet interiors preserve a unique record from the formation of the Solar System 4.6 billion years ago. Reaching that information was one of the key tasks of Rosetta and Philae. There were two principal experiments designed to 'see' inside the comet's nucleus: the COmet Nucleus Sounding Experiment by Radio-wave Transmission (CONSERT) instrument and the Radio Science Investigation (RSI).
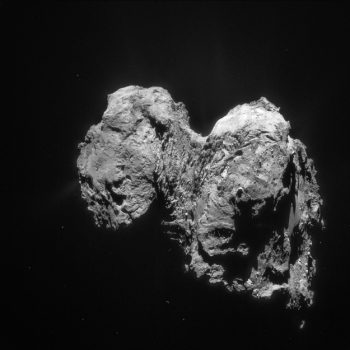
Image taken with the navigation camera (NavCam), 28 January 2016. Credit: ESA/Rosetta/NAVCAM, CC BY-SA IGO 3.0
Comets are known to be mixtures of dust and ice. Yet puzzlingly, measurements show that 67P/C-G's density is much lower than ice. This means the comet has a high porosity, and could be an indicator that there are huge empty caverns inside it. However, neither CONSERT nor RSI find any evidence to support this.
Instead, the investigations have shown that the porosity comes about because the comet is a more or less uniform mixture of ice and 'fluffy' dust grains, rather than a honeycomb structure featuring large voids.
CONSERT works by 'sounding' the comet's nucleus. This involves beaming radio waves through the comet from Philae to the orbiter.
"We know the characteristics of the signal used for the sounding. After propagation through the nucleus the signal is received by Rosetta and the way it has been modified provides information about the nucleus' interior," says Valérie Ciarletti, LATMOS, Guyancourt, France, and co-investigator on CONSERT.
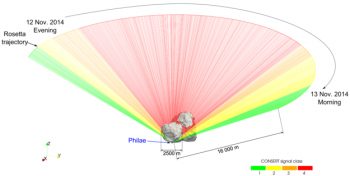
This diagram shows the propagation of signals between Rosetta and Philae through the nucleus of Comet 67P/Churyumov-Gerasimenko, between 12 and 13 November 2014. Green represents the best signal quality, decreasing in quality to red for no signal. Credit: ESA/Rosetta/Philae/CONSERT
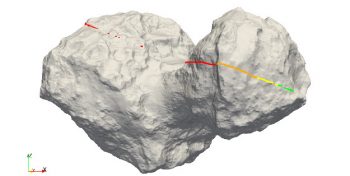
This image shows the path on the comet’s surface where waves transmitted by Philae during the First Science Sequence, between 12 and 13 November 2014, emerged from the nucleus. Green represents the best signal quality, decreasing in quality to red for no signal. Credit: ESA/Rosetta/Philae/CONSERT
As the waves pass through the comet they are slowed down by the ice and dust they encounter. When they are detected by Rosetta, the delay in their transmission and the way the radio pulse's shape has changed allows the team to compare the results with theoretical models of the comet interior to see which fits the data best.
This work shows that the comet's low density might be an intrinsic property of the material that makes up the interior. For example, instead of being a compacted solid, the dust in the interior is 'fluffy'. This fits with the kind of dust grains that Rosetta's COSIMA and GIADA instruments have detected being given off by the comet.
CONSERT's results are backed up by those of RSI. Situated on Rosetta, the instrument measures the distribution of mass inside the comet based upon the way the comet's gravity pulls the orbiting spacecraft. The movement is measured by changes in the frequency of the spacecraft's signals when they are received at Earth.
It is a manifestation of the Doppler effect, which is produced whenever there is movement between a source and an observer. This same effect causes emergency vehicle sirens to change pitch as they pass by.
The variations in Rosetta's signals were analysed to give a picture of the gravity field across the comet. Large internal caverns would have been noticeable by a tell-tale drop in the spacecraft's acceleration. Again, no such signal was recorded.
What does the comet's shape tell us?
When the strange shape of the comet was first seen by Rosetta, scientists began asking whether the two lobes of 67P/C-G started as two different comets that collided at low speed and stuck together, or if it is the result of erosion caused by the nucleus activity trigged when the nucleus gets closer to the Sun.
Images from the OSIRIS camera show the two lobes appear very different and so are almost certainly two different bodies. On-going analysis with CONSERT data is further investigating this idea.
The instrument was active during Philae's descent and the scientists measured not only the direct signal between Philae and Rosetta, but also its reflection off the surface of both lobes. Current work is aiming at an accurate analysis of the reflection signal. This will allow the surface properties of each lobe to be compared, which in turn will provide further insight into whether or not these two lobes originated as separate entities.
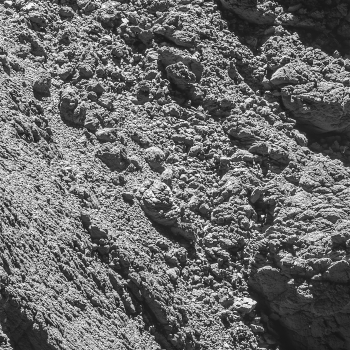
The lander was identified at the far right of the image, just above centre. Credit: ESA/Rosetta/MPS for OSIRIS Team MPS/UPD/LAM/IAA/SSO/INTA/UPM/DASP/IDA
Now that Philae's location is known, it confirms the scientists' suspicion that all CONSERT data received so far has been propagating only through the comet's smaller lobe. This could well be because Philae came to rest on its side and so the antenna was not in the expected orientation, namely sitting horizontal to the surface. Being on its side has meant that there is a mismatch between the antennas on Philae and Rosetta, hence the signal was probably not strong enough to pass through the bulk of the larger lobe as well.
"Now that we know precisely where Philae is and, most of all, its attitude as well as the very close environment, we are able to simulate the whole radio link from Philae to Rosetta. We might understand if the antenna's configuration is the explanation for the non-detection or if we need to also consider attenuation inside the larger lobe’s nucleus to explain why we did not detect any signal," says Ciarletti.
Probing the surface layers
The effect of the Sun's heat has had a major impact at the surface layer of the comet. Philae has already shown that the surface of the small lobe is different from the interior. The propagation of the radio waves from CONSERT is driven by the permittivity of the nucleus, which is an electrical property governed by the comet’s composition, porosity and temperature. Philae also measured the surface permittivity using its Surface Electric Sounding and Acoustic Monitoring Experiment Permittivity Probe (SESAME-PP) instrument.
This shows that at Abydos, the location where Philae has come to rest, the top metre of the surface has a significantly higher permittivity than the interior, which is consistent with a lower porosity at the surface. This indicates that the surface layers are more compacted than the interior.
There is even more science to come as Rosetta is guided to its controlled impact with the comet on 30 September. The radio link to Earth will mean that RSI continues collecting data right to the very end. The closer the spacecraft gets the comet, the deeper in its gravity field it will be and the better the precision of the readings.
Thanks to the Rosetta and Philae mission we now know 67P/C-G better than any other comet – both inside and out.
--
Insights from the Rosetta mission into what lies beneath the comet's surface were presented by Valérie Ciarletti during today's science briefing at the European Space Operations Centre (ESOC) in Darmstadt, Germany.
---
COMET LANDSCAPES AND MAPS OF THE SOUTHERN HEMISPHERE
During today's science briefing at ESA's ESOC, Mohamed El-Maarry (University of Bern) presented a series of highlights about the landscape of Comet 67P/Churyumov-Gerasimenko. Here are some highlights from his presentation.
Over the past two years, Rosetta mapped the entire surface of the comet at high-resolution, resolving very small features and monitoring surface changes with time.
The comet nucleus is indeed very dark (see our 2014 blog post NAVCAM's shades of grey), reflecting only about 4% of the light that hits it. But why is it so dark?
Measurements from both Rosetta and Philae report that most of the surface is dry (with the exception of icy boulders) and covered in organic material, most of which had never been detected on the surface of a comet (on this topic, see previous blog posts: Extremely dark, dry and rich in organics and Science on the surface of a comet).
The surface presents a rich diversity in texture, with the northern hemisphere mostly covered in dust, produced by the comet's activity – for example in the Ma'at and Ash regions.
Philae's multiple landings allowed scientists to observe in details two distinct regions on the comet's surface: the smooth-covered terrains at the first touchdown point, Agilkia, and the hard surface at its final resting point, Abydos.
Some of the smooth material, for example in Imhotep, also gives rise to the most dynamic features that have been observed on the comet so far. Surface changes have been observed around the time of perihelion (see the 2015 post Comet surface changes before Rosetta’s eyes).
Finally, a look at the southern hemisphere, which could not be observed until mid-2015 and gradually became illuminated shortly before the comet's perihelion. The surface morphology of the southern hemisphere is very different, showing a clear dichotomy with its northern counterpart mainly because of the absence of wide-scale smooth terrains, dust coatings and large unambiguous depressions.
This is likely caused by the uneven distribution of seasons on the comet. Due to the double-lobed shape of 67P/C-G and the inclination of its rotation axis, the northern hemisphere experiences a very long summer, lasting over 5.5 years, while the southern hemisphere undergoes a long, dark and cold winter.
However, a few months before the comet reaches perihelion – the closest point to the Sun along its orbit – the situation changes, and the southern hemisphere transitions to a brief and relatively hot summer.
As the southern summer is much shorter and more intense than in the northern hemisphere, the erosion is higher there by a factor of 3, giving rise to flattened out features. Scientists believe that, due to the more intense activity, dust is ejected at higher speed and it is either lost to space or falls back on the northern hemisphere (read more about the dust transfer across the comet in the recent blog post The surprising comet).
Below are a series of maps highlighting the various regions on the southern hemisphere of Comet 67P/C-G:
And finally a set of views of regions across the entire comet, both in the northern and southern hemisphere:
--
This blog is based on the presentation by Mohamed El-Maarry during today's science briefing at the European Space Operations Centre (ESOC) in Darmstadt, Germany.
The images of the southern hemisphere and the comet maps are from the paper 'Regional surface morphology of comet 67P/Churyumov-Gerasimenkofrom Rosetta/OSIRIS images: The southern hemisphere' by El Maarry et al, which was published earlier this year in Astronomy and Astrophysics.
Quelle: ESA