.
NASA has selected eight technology proposals for investment that have the potential to transform future aerospace missions, introduce new capabilities, and significantly improve current approaches to building and operating aerospace systems.
Awards under Phase II of the NASA Innovative Advanced Concepts (NIAC) Program can be worth as much as $500,000 for a two-year study, and allow proposers to further develop concepts funded by NASA for Phase I studies that successfully demonstrated initial feasibility and benefit.
“The NIAC program is one of the ways NASA engages the U.S. scientific and engineering communities, including agency civil servants, by challenging them to come up with some of the most visionary aerospace concepts,” said Steve Jurczyk, associate administrator of NASA’s Space Technology Mission Directorate in Washington. “This year’s Phase II fellows have clearly met this challenge.”
Phase II studies allow awardees to refine their designs and explore aspects of implementing the new technology. This year’s Phase II portfolio addresses a range of leading-edge concepts, including: an interplanetary habitat configured to induce deep sleep for astronauts on long-duration missions; a highly efficient dual aircraft platform that may be able to stay aloft for weeks or even months at a time; and a method to produce “solar white” coatings for scattering sunlight and cooling fuel tanks in space down to 300 °F below zero, with no energy input needed.
The selected concepts are:
Advancing Torpor Inducing Transfer Habitats for Human Stasis to Mars, John Bradford, Space Works, Inc. in Atlanta
Cryogenic Selective Surfaces, Robert Youngquist, Kennedy Space Center in Florida
Directed Energy Interstellar Study, Philip Lubin, University of California, Santa Barbara
Experimental Demonstration and System Analysis for Plasmonic Force Propulsion, Joshua Rovey, University of Missouri in Rolla
Flight Demonstration of Novel Atmospheric Satellite Concept, William Engblom, Embry-Riddle Aeronautical University in Daytona Beach, Florida
Further Development of Aperture: A Precise Extremely Large Reflective Telescope Using Re-configurable Elements, Melville Ulmer, Northwestern University in Evanston, Illinois
Magnetoshell Aerocapture for Manned Missions and Planetary Deep Space Orbiters, David Kirtley, MSNW, LLC in Redmond, Washington
Tensegrity Approaches to In-Space Construction of a 1g Growable Habitat, Robert Skelton, Texas Engineering Experiment Station in La Jolla, California
NASA selected these projects through a peer-review process that evaluated innovativeness and technical viability.
“Phase II decisions are always challenging, but we were especially challenged this year with so many successful Phase I studies applying to move forward with their cutting-edge technologies,” said Jason Derleth, the NIAC program executive at NASA Headquarters in Washington. “Whether it's tensegrity habitats in space, new ways to get humans to Mars, delicate photonic propulsion, or any one of the other amazing Phase II studies NIAC is funding, I'm thrilled to welcome these innovations and their innovators back to the program. Hopefully, they will all go on to do what NIAC does best - change the possible.”
All projects are still in the early stages of development, most requiring 10 or more years of concept maturation and technology development before use on a NASA mission.
NIAC is funded by NASA’s Space Technology Mission Directorate, which innovates, develops, tests, and flies hardware for use in NASA’s future missions. Through programs such as NIAC, the directorate is demonstrating that early investment and partnership with scientists, engineers and citizen inventors from across the nation can provide technological dividends and help maintain America's leadership in the new global technology economy.
-
Light Weight Multifunctional Planetary Probe for Extreme Environment Exploration and Locomotion
.
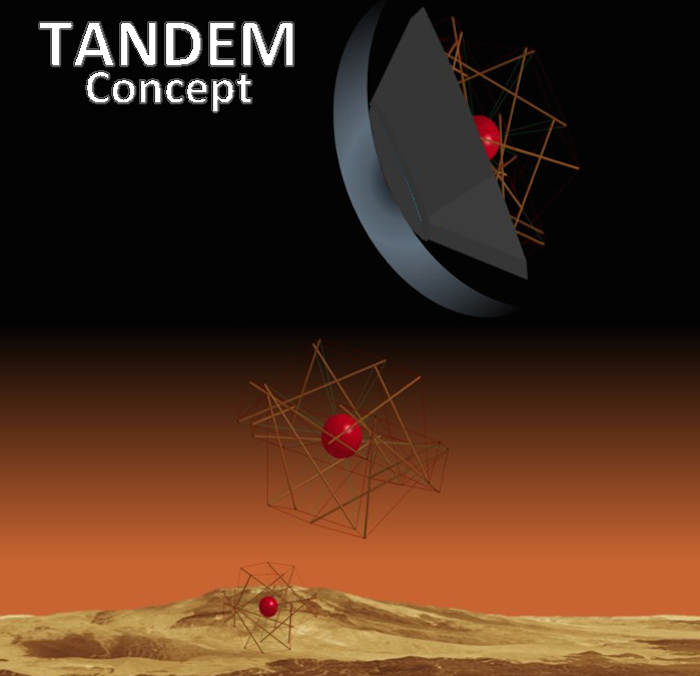
Artist’s rendering of the TANDEM concept showing a deployable heat shield and tensegrity structure for high risk landing zones for extreme environmental missions.
Virginia Polytechnic Institute and State University
Description
TANDEM concept
The Tension Adjustable Novel Deployable Entry Mechanism (TANDEM) is a specially configured tensegrity structure that is designed to act as the frame of a deployable heat shield. TANDEM combines the infrastructure used for the Entry, Decent, and Landing sequence as well as on-the-ground locomotion into a single multifunctional system. Reusing the same infrastructure for every section of the mission makes TANDEM one of the most efficient systems ever proposed. Although its touchdown mass may be higher than other landers, the versatility of its components translates to a tremendous mass reduction for the whole mission. TANDEM is an entry vehicle and lander for planetary exploration class probes. It utilizes a semi-rigid, 3-D woven carbon-cloth deployable heat shield. What separates TANDEM from other deployable entry vehicles is its use of tensegrity structure as the frame of the deploying mechanism. The use of tensegrity robotics for entry vehicles is a currently unexplored concept, providing numerous potential benefits during entry and descent. These benefits include a mass efficient mechanism for actively guided entry, active control of L/D ratio, as well as active control of aerodynamic center. Like most tensegrity concepts, it inherently provides omni-directional protection on impact. However, due to the unique shape of the vehicle, it can actively adjust its configuration for optimal landing. If unforeseen circumstances cause the lander to turn upside-down, TANDEM can be programed to change its aerodynamic center and reorient itself. Alternatively, it can adjust its outer circumference to provide a safer landing based on its free fall orientation. This adds a remarkable level of reliability and safety to any mission using the TANDEM concept. Conventional tensegrity locomotion depends largely on actuation of the outer cables. This requires mechanical devices in each strut to reel in the cables. However, for extreme environment applications such a system can prove infeasible as it requires each strut to be protected from the environment. This in turn can cause the lander to be prohibitively heavy. On the other hand, the TANDEM technology can be effectively applied for locomotion through using only inner cable actuation. The locomotion mechanisms can hence be housed in a central payload module, using a common insulation and pressure vessel. TANDEM is designed to enable landing at any orientation and can traverse significantly rougher terrain than existing rovers. This means new landing sites can be reached. Instead of landing in low risk areas then traveling to the closest area of scientific interest, missions using TANDEM can land directly in the region of interest. The TANDEM concept provides a high level of adaptability and controllability which can be utilized in entry, descent, landing, and for locomotion by actuating specific inner cables. This opens all new avenues in utilizing multifunctional exploration systems with enhanced maneuvering options. Additionally, TANDEM presents a robust, low weight method of locomotion that does not require ultra-high temperature mechanisms for mobility in extreme environments. These attributes make it a superior concept for near- to mid-term missions.
-
Venus Interior Probe Using In-situ Power and Propulsion (VIP-INSPR)
.
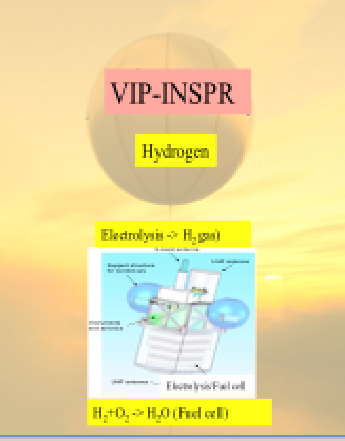
We envision a novel architecture for Venus Interior Probes based on in-situ resources for power generation (VIP-INSPR). Proposed Venus probe is based on the generation of hydrogen from electrolysis at high altitudes using solar array, storing it in chemical hydride, utilizing it for altitude control in a balloon system and for power generation in fuel cell at lower altitudes. It is a challenge to have a durable power source in the low altitude environments, due to low solar intensity and low efficiency of RTG is inefficient. Primary batteries survive only for 1-2 hours. VIP-INSPR will refill hydrogen on one end and provide power on the other side continuously, thus enabling sustained exploration of the Venus atmosphere.
-
Reconstituting Asteroids into Mechanical Automata
.
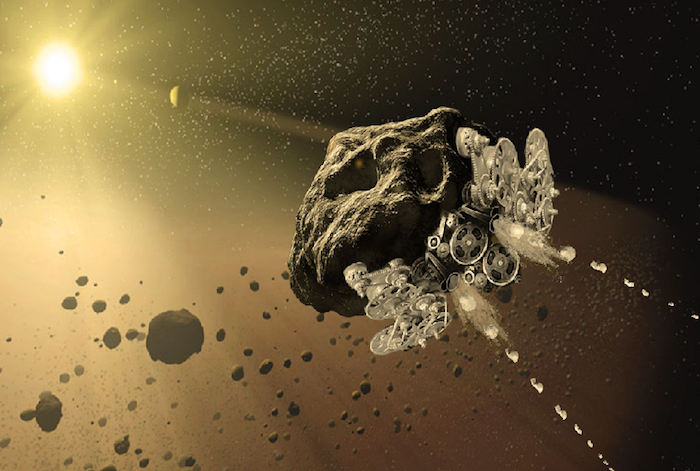
The objective of this study is for Made In Space (MIS) to establish the concept feasibility of using the age-old technique of analog computers and mechanisms to convert entire asteroids into enormous autonomous mechanical spacecraft. Project RAMA, Reconstituting Asteroids into Mechanical Automata, has been designed to leverage the advancing trends of additive manufacturing (AM) and in-situ resource utilization (ISRU) to enable asteroid rendezvous missions in which a set of technically simple robotic processes convert asteroid elements into very basic versions of spacecraft subsystems (GNC, Propulsion, Avionics). Upon completion, the asteroid will be a programmed mechanical automata carrying out a given mission objective; such as relocation to an Earth-Moon libration point for human rendezvous. This technique will create an affordable and scalable way for NASA to achieve future roadmap items for both the Human Exploration and Operations Mission Directorate (HEOMD) and the Science Mission Directorate (SMD) such as Asteroid Redirect Mission (ARM), New Frontiers Comet Surface Sample Return, and other Near Earth Object (NEO) applications. It is estimated that an order of magnitude increase in NEO targets can be explored for the same mission cost with the RAMA approach compared to the SOA Asteroid Redirect Mission (ARM) architecture by removing the need to launch all spacecraft subsystems and instead converting the asteroid into them in-situ. Assuming the development trends continue for industry based AM methods as well as NASA and industry investments in ISRU capabilities, Project RAMA will create a space mission architecture capable of achieving the aforementioned NASA goals within a 20-30 year time frame. Furthermore, as described in the proposal, the identified study path will provide insight into near term Mission 'Pull' technologies worth investment in order to create the development roadmap for the proposed 'Push' technologies for achieving NASA's long term strategic goals.
-
Mars Molniya Orbit Atmospheric Resource Mining
.
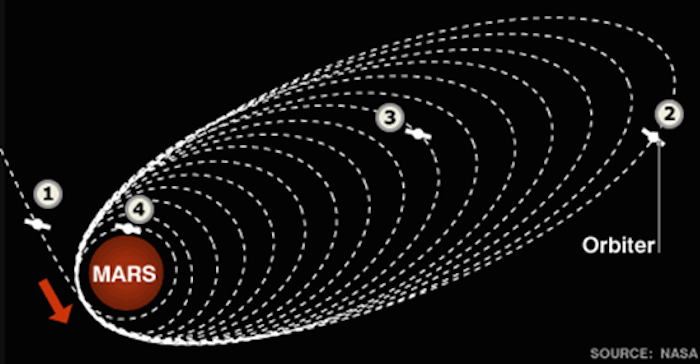
Mars planetary surface access is one of NASA's biggest technical challenges involving advanced entry, descent, and landing (EDL) technologies and methods. This NASA Innovative Advanced Concept (NIAC) project intends to solve one of the top challenges for landing large payloads and humans on Mars by using advanced atmospheric In-Situ Resource Utilization (ISRU) methods that have never been tried or studied before. The proposed Mars Molniya Orbit Atmospheric Resource Mining concept mission architecture will make Mars travel routine and affordable for cargo and crew, therefore enabling the expansion of human civilization to Mars.
While technology studies have been performed considering new approaches to land larger mass payloads on Mars, a concept that integrates this EDL objective with recent advances demonstrated in supersonic retro-propulsion and in-situ resource technology has not been considered. This novel system, and associated concept of operations, offers a solution for large mass EDL (>18 t) using retro-propulsion methods with ISRU propellants made on orbit.
-
Journey to the Center of Icy Moons
.
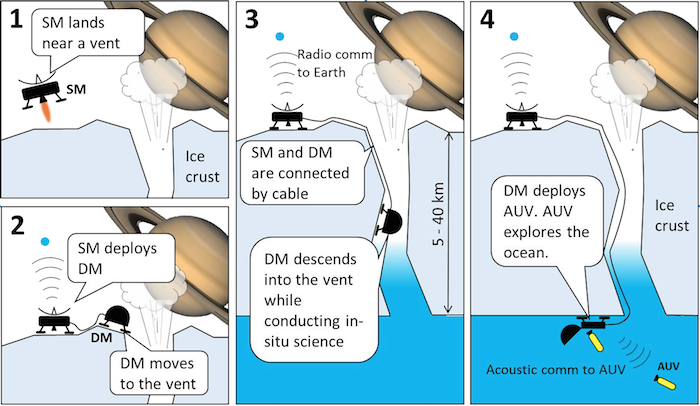
In Jules Verne’s classic science fiction, Journey to the Center of the Earth, Professor Otto Lidenbrock and his company descend into an Icelandic volcano to explore it in the name of science, discover a vast subterranean ocean among other unexpected wonders, and must resiliently survive the experience to complete their mission. This is exactly what we want to do in reality on Europa and Enceladus. Several concepts have already been studied to explore these moons’ subsurface oceans using autonomous underwater vehicles (AUVs). However, access to subsurface ocean remains to be an outstanding challenge. The proposed concept is to deploy a surface-to-subsurface robotic system, namely Icy-moon Cryovolcano Explorer (ICE), which will land on the surface of an icy moon, traverse to a cryovolcano, descend into its opening, perform in-situ science in the vent or crevasse, and ultimately deploy underwater vehicles to explore a subsurface ocean.
ICE involves three modules: Descent Module (DM), Surface Module (SM), and AUVs. DM carries AUVs and descends into a vent by using a combination of roving, climbing, rappelling, and hopping, like an experienced human alpinist. The estimated gas density of an ejecting plume is sufficiently low, therefore its dynamic pressure (< 1 Pa) would not be an obstacle for descent. SM stays on the surface, generates power by RTG and/or solar cells, and communicates with Earth. DM relies on the power and communication link provided by SM through a cable to minimize the size and weight. It is a highly autonomous agent being capable of quickly responding to a dynamically changing environment, such as episodic eruption, and resiliently handling any anomalies under significant communication latency. Once DM reaches the subsurface ocean, it launches the AUVs to explore the exotic environment that potentially harbors life.
ICE brings three unique benefits. First, it enables in-situ science in a cryovolcano vent. Although orbiters can perform in-situ science of plumes, relatively large (up to 1 μm) dust grains are hard to reach orbital altitude. Yet it is those mineral grains that carry rich information about the habitability of the subsurface ocean. Second, ICE enables the exploration of subsurface oceans by providing an access to it. Third, it enables the operation of AUVs in subsurface ocean by providing three essential services: communication, localization, and power. Since water blocks radio waves, communication and localization are particularly significant challenges for AUVs. DM of ICE communicates with AUVs though acoustic communication. DM then transfers the data though an optic cable to SM, from which the data is transmitted to Earth by radio. DM also sends an acoustic localization beacon and serves as a battery charging station, potentially unnecessitating RTG on the AUV.
In the proposed study we will (1) develop mission concepts for ICE, (2) identify the primary risks associated with the mission, and identify potential mitigations for these risks, and (3) perform a feasibility analysis for the mission, which will include performing several system trades, including one focused on the hardware platform (e.g., climbing robot vs. repelling robot vs. hovering robot), and another one focused on the autonomy software capabilities (with the goal to identify the appropriate scope of the autonomous functionality required to execute the mission concepts). These tasks will result in identifying driving requirements for the system, including candidate science targets, power needs, resilience needs, etc. In summary, we will develop a concept for ICE that elaborates and refines the science and exploration benefits descried above, and we will analyze the benefits and risks associated with realizing this concept. A successful completion of the project will mature this exciting concept into a credible element of the growing outer planets and icy moons exploration portfolio.
-
Fusion-Enabled Pluto Orbiter and Lander
.
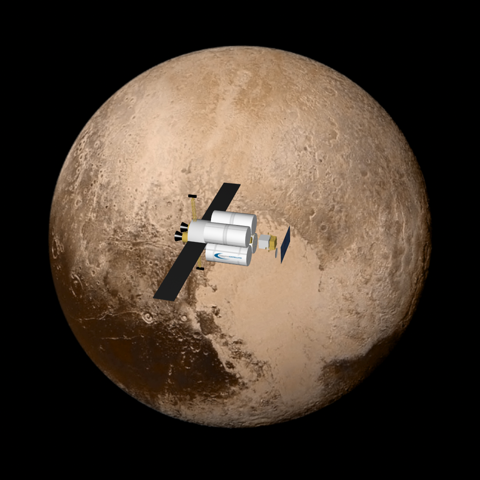
The Direct Fusion Drive (DFD) concept provides game-changing propulsion and power capabilities that would revolutionize interplanetary travel. DFD is based on the Princeton Field-Reversed Configuration (PFRC) fusion reactor under development at the Princeton Plasma Physics Laboratory. The mission context we are proposing is delivery of a Pluto orbiter with a lander. The key objective of the proposal is to determine the feasibility of the proposed Pluto spacecraft using improved engine models. DFD provides high thrust to allow for reasonable transit times to Pluto while delivering substantial mass to orbit: 1000 kg delivered in 4 to 6 years. Since DFD provides power as well as propulsion in one integrated device, it will also provide as much as 2 MW of power to the payloads upon arrival. This enables high-bandwidth communication, powering of the lander from orbit, and radically expanded options for instrument design. The data acquired by New Horizons' recent Pluto flyby is just a tiny fraction of the scientific data that could be generated from an orbiter and lander. We have evaluated the Pluto mission concept using the Lambert algorithm for maneuvers with rough estimates of the engine thrust and power. The acceleration times are sufficiently short for the Lambert approximation, i.e. impulsive burns, to have some validity. We have used fusion scaling laws to estimate the total mission mass and show that it would fit within the envelope of a Delta IV Heavy launch vehicle. Estimates of the amount of Helium 3 required to fuel the reactor are within available terrestrial stores.
In this Phase I study, we propose to analyze the Pluto mission concept using new models of the engine. We will develop an optimal trajectory including limits on the thrust steering and range of throttle. The throttling of the thrust and specific impulse will affect the efficiency, which we have not yet attempted to model.
Direct Fusion Drive is a unique fusion engine concept with a physically feasible approach that would dramatically increase the capability of outer planet missions. The fusion-enabled Pluto mission proposed here is credible, exciting, and the benefits to this and all outer planet missions are difficult to overstate. The truly game-changing levels of thrust and power in a modestly sized package
could integrate with our current launch infrastructure while radically expanding the science capability of these missions.
-
Advancing Torpor Inducing Transfer Habitats for Human Stasis to Mars
.
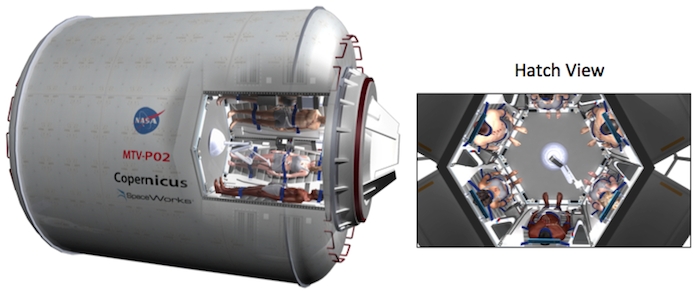
SpaceWorks proposes the development of an advanced habitat system for transporting crews between the Earth and Mars. This new and innovative habitat design is capable of cycling the crew through inactive, non-cryonic torpor sleep states for the duration of the inspace mission segments. Under this effort, SpaceWorks will (i) Expand the Phase I medical team to address key challenges identified in the initial effort, (ii) Examine key habitat engineering aspects to further explore and refine design and identify further potential performance gains, (iii) initiate validation studies with leading medical researchers to understand the effects of prolonged hypothermia, and (iv) Consider the technology’s impact on alternate exploration missions (Mars moons, asteroid belt, Jovian and Saturn system, etc.).
.
Magnetoshell Aerocapture for Manned Missions and Planetary Deep Space Orbiters
.
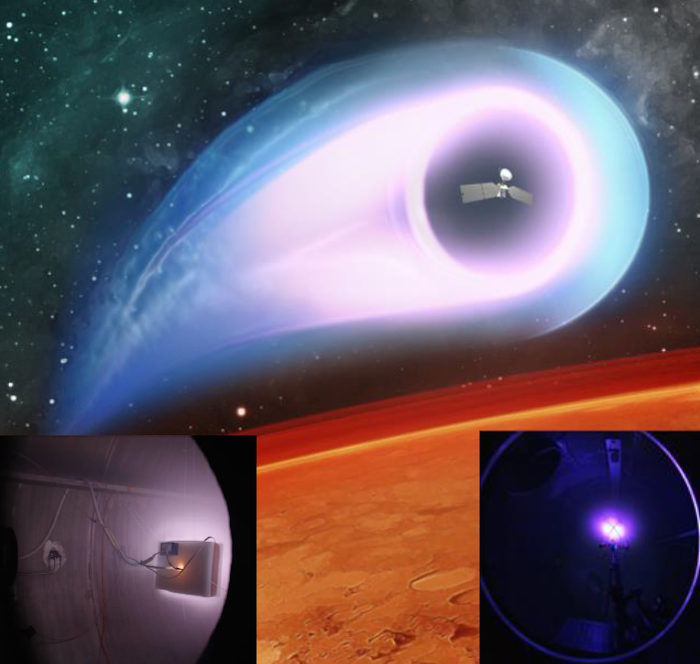
It is clear from past mission studies that a manned Mars mission, as well as deep space planetary orbiters will require aerobraking and aerocapture which use aerodynamic drag forces to slow the spacecraft. Aerocapture would enable long term studies of the outer planets and their moons that would not be possible with existing braking technologies. While utilizing planetary atmospheres to slow down and capture spacecraft would dramatically reduce the cost, launch mass, and travel time, current technologies require significant additional spacecraft mass and risk, as the spacecraft must descend deep into a planetary atmosphere that is not well characterized in order to produce significant drag on a relatively small, fixed dimension aeroshell or temperature and structurally sensitive inflatable ballute.
The Magnetoshell deploys a simple dipole magnetic field containing a magnetized plasma. It is interaction of the atmosphere with this magnetized plasma that supplies a significant impediment to atmospheric flow past the spacecraft, and thereby producing the desired drag for braking. Frictional heating would no longer be of concern as the energy dissipation required to slow the spacecraft would be deposited into the plasma ions helping to maintain the Magnetoshell plasma while at the same time shielding the spacecraft itself from frictional heating. With the aeroshell now being composed of massless magnetic field, the transverse scale of the magnetic barrier can be as large as 100 meters while requiring no more than a gram of plasma. With the ability to rapidly and precisely modify the drag in varying atmospheric conditions, much larger forces can now be achieved at low risk, enabling very aggressive aerocapture maneuvers. By providing power in a pulsed manner, the thermal and power processing requirements can be kept modest and with conventional technologies.
In Phase I a full system was designed for Neptune and Mars missions. This analysis showed that a 200 kg, 2 m magnet could generate a 9 m radius Magnetoshell for Neptune aerocapture with a 21 km/s injection at a peak force of 150 N entirely removing the need for a TPS. At Mars, a 2.5 m magnet could generate a 21 meter radius Magnetoshell, providing aerocapture for a 60 metric ton payload removing the dedicated aerocapture TPS and saving $2 B for DRA 5.0. A transient analytic model was developed evolving the radial plasma parameters for a variety of plasma, neutral, and magnetic parameters. Finally, a stationary 1.6 meter argon Magnetoshell was fully demonstrated and a 1000:1 increase in aerodynamic drag was found. This experimental program definitively demonstrated a subscale Magnetoshell by eliminating electromagnetic interference, utilizing a dielectric torsional thrust stand, and placing all key electrical components under vacuum in the plasma environment. In addition, by decrease the dynamic pressure requirements while simultaneously shielding the spacecraft, heating during an Aerocapture maneuver could be reduced by 10,000X. In the proposed Phase II, the complete mission benefits of the Magnetoshell system will be proven. During this Phase II detailed mission studies will investigate the 3D orbital entry and capture mechanics while investigating the thermal, structural, electrical, and magnetic requirements of a Magnetoshell system. These studies will determine the risk, cost, and overall benefits of a full scale Magnetoshell aerocapture system for a range of science and manned missions to the inner and outer solar system. A hypersonic plasma-neutral interaction validation study will prove the fundamental particle and plasma effects at 10 to 15 km/s velocities and relevant densities. And finally, a detailed Aerocapture orbital gravity model, based on the satellite tour design program (STOUR) will be developed to model in 3D, the full dynamics of an interplanetary, 60 meter Aerocapture system.
-
Tensegrity Approaches to In-Space Construction of a 1g Growable Habitat
.
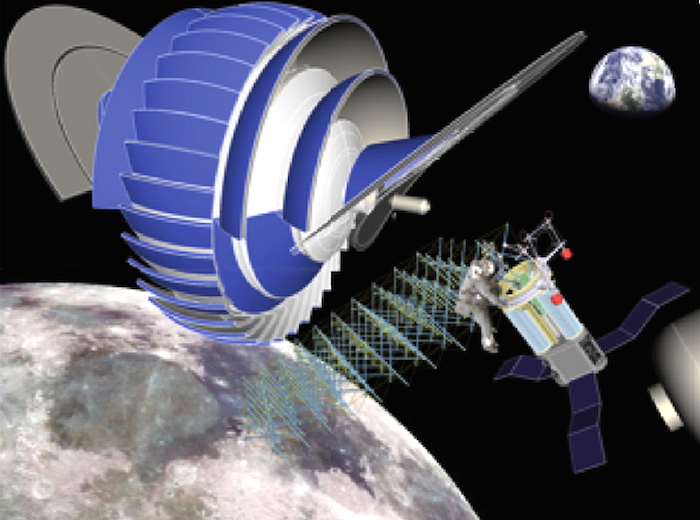
This proposal seeks to design a rotating habitat with a robotic system that constructs the structure and provides a habitat growth capability. The tensegrity technology allows minimum mass of both the habitat and the robotic system. This proposal solves three unsolved space travel problems: a) growth, b) radiation protection, and c) gravity. Our innovative tensegrity-based evolvable habitat designs will solve three critical technical problems that NASA must address: the biological effects of microgravity on humans in long duration space flight, the long duration biological effects of ionizing radiation on human physiology in deep space (beyond the Earth’s magnetosphere) and, the need for outposts in deep space to evolve dynamically over time as mission needs grow. Our technologies fit hand in glove with NASA's new strategy and will be a key enabler in making NASA's vision of pioneering the space frontier a reality because they are the only economically feasible approach to building habitats that can grow, spin, and manufacture in space. This NIAC Phase II effort will perform critical proof of concept studies, analysis, and ground demonstrations to prove the feasibility of Growth-Adapted Tensegrity Structures (GATS) and their benefit for NASA's evolvable proving ground approach to human exploration. As part of this effort we will conduct mission studies showing how a version 1.0 outpost in Lunar Distance Retrograde Orbit (LDRO) made from GATS can grow and evolve while utilizing asteroid resources for radiation shielding and later integrate with an asteroid ISRU system such as the NIAC funded ApisT M architecture proposed by Sercel. We will perform mission analysis in collaboration with Sercel showing how asteroid regolith slag left over from the production of rocket propellant can be accumulated over time in LDRO and integrated as radiation shielding into the structure of the GATS based evolvable outpost thereby saving many billions of dollars in launch costs and helping to make NASA’s program of human exploration more affordable. The critical enabling technology for our GATS technology is Tensegrity Engineering, where new design methods, new dynamic models, and new control approaches are specialized for networks of axially-loaded elements, allowing the structural mass to be minimized, while the dynamic response can be controlled with minimal energy, with repairable, growable structural methods, using tractable analytical tools that are now available. We also capitalize on asteroid resources for radiation shielding of our habitat. This NIAC Phase II effort will perform critical proof of concept studies, analysis, and ground demonstrations to prove the feasibility of Growth-AdaptedTensegrity Structures (GATS) and their benefit for NASA's evolvable proving ground approach to human exploration.
.
Further Development of Aperture: A Precise Extremely Large Reflective Telescope Using Re-configurable Elements
.
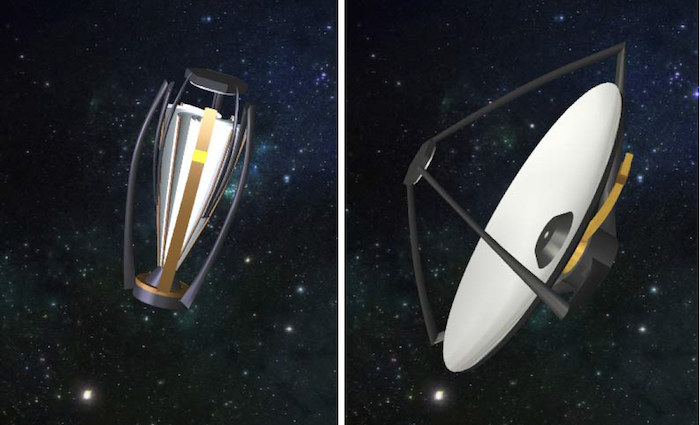
One of the pressing needs for space ultraviolet-visible astronomy is a design to allow larger mirrors than the James Webb Space Telescope primary. The diameter of the rocket fairing limits the mirror diameter such that all future missions calling for mirrors up to 16 m in diameter or larger will require a mirror that is deployed post-launch. In response to the deployment requirement, we address the issues of this concept called "A Precise Extremely large Reflective Telescope Using Reconfigurable Elements (APERTURE) with both hardware experiments and software simulations. APERTURE will use a deployable membrane-like mirror. The mirror figure will be corrected after deployment to bring it into better or equal lambda/20 deviations from the prescribed mirror shape, where lambda (typically 400 nm-1 micron) is the operational wavelength. Instead of using the classical piezoelectric-patch technology, our concept is based on a continuous coating of a Magnetic Smart Material (MSM). We expect that the initially deployed mirror will not have a perfect figure. Thus our design uses magnetic write heads to produce stress in the MSM and improve the figure, post deployment. This Phase II NIAC proposal is to address two of the tall poles in the concept: (a) Can corrections on a large size be made and retained for a long enough time (> 1 week); (b) Can deployment be done in such a way that the figures corrections are small enough to be correctable via the MSM plus magnetic field, and at the same time, the in plane stresses as small enough to allow the stresses resulting magnetic field injected into the MSM plus magnetic fields to make the necessary corrections. Tall pole “a” will be primarily the responsibility of Northwestern University (NU) and “b” of University of Illinois Urbana-Champaign. NU will carry out overall scientific leadership and will coordinate and solicit input from GSFC, JPL, and NIST.
.
Cryogenic Selective Surfaces
.
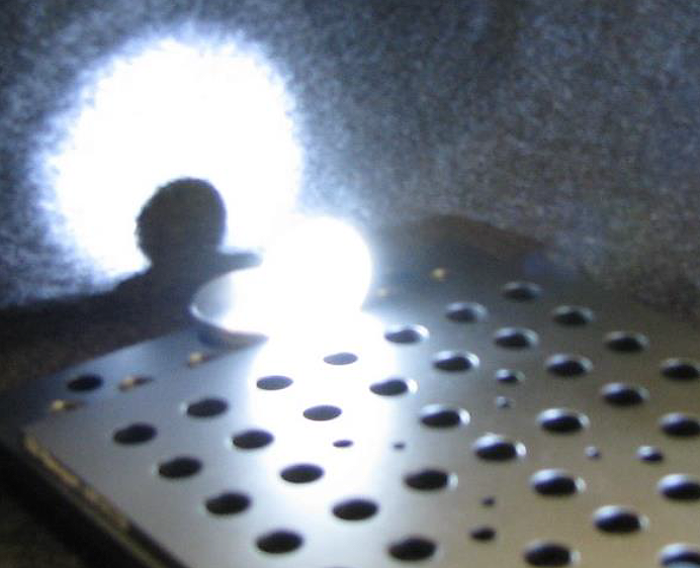
During our Phase 1 NIAC study we discovered a novel coating we call “Solar White” that, when used in deep space, is predicted to reflect more than 99.9% of the sun’s energy. We have shown analytically that a sphere covered with a 10 mm thick coating of Solar White and located far from the Earth and at 1 Astronomical Unit from the Sun can achieve a steady state temperature below 50 K, the freezing point of oxygen. The ramifications of such a coating are broad and significant, ranging from enabling long-term cryogenic storage to allowing passive high temperature superconductor operation in space. However, the development of these coatings is only at a theoretical modeling stage. The next two significant steps required to advance this breakthrough technology are to fabricate these coatings and test their performance. Construction of rigid versions of the coatings may be difficult, because they are composed of only one material deposited onto a metallic reflector, though there are precedents such as rigid foam and Space Shuttle Tiles to use as models. We have access to chemistry and materials experts who will work on constructing the coatings and we will liaise with Ames Research Center to help leverage the expertise available at that NASA center. Testing of our algorithms can be performed in parallel because we can construct non-rigid versions of the Solar White Coating consisting of powdered materials sandwiched between a mirror and a cover slide as a test sample. Testing would occur in a simulated deep space environment, which we will create using a vacuum chamber, a 20K cryo-cooler, and an irradiance source. By monitoring the difference in steady-state temperature in the test sample with and without irradiated energy and comparing this against our algorithmic predictions will be able to refine our models of these novel coatings when exposed to solar and infrared irradiance. We have shown in our Phase I effort that the use of Solar White could theoretically allow liquid oxygen to be maintained on a long duration mission to Mars. The Solar White coating does this by reducing not only solar heating, but also radiative heating from other, warmer, portions of the Mars vehicle and conductive heating along support struts and flow lines. We now propose to extend the deep space cryogenic use of this exciting new coating by examining the storage of LOX on the Moon. We will also examine the use of Solar White on a LOX/LN2 deep space storage depot and determine the possible locations of this depot relative to infrared emitters such as planets and moons, as well as the Sun and nearby space vehicles.
-
Quelle: NASA
4110 Views