.
the more modern, larger values of 1.5–1.7 AU (e.g., Kasting et al., 1993; Kopparapu et al., 2013).
Since the temperature-dependent carbonate-silicate cycle provides a negative feedback, it could have been responsible for the long-term stabilization of Earth’s surface temperature. With a sufficiently high silicate weathering rate, even a lifeless planet could remain habitable. However, since temperature- dependent silicate weathering requires sub-aerial weathering of silicate rocks (either granitic or basaltic), the magnitude of the negative feedback of silicate weathering is roughly pro- portional to the amount of sub-aerial continental crust. In the first billion years of Earth’s history, the fraction of the surface of Earth where sub-aerial erosion would have been possible may have been extremely small (Flament et al., 2008; Abbot et al., 2012; Dhuime et al., 2015). Flament et al. (2008) modeled the sub-aerial weathering as a function of time and estimated that in the late Archean (*2.5Gya) 2–3% of the Earth’s surface was sub-aerial continent.
The little continental crust present was largely submerged. Thus, it is likely that early in Earth’s history the negative feedback of the carbonate-silicate cycle may have been inop-
erative or at least significantly less effective than today. This undermines the main negative feedback mechanism proposed to stabilize surface temperature on wet rocky planets for the first billion years or so, when they are most likely to experience runaway greenhouse or runaway glaciation due to high in- ventories of primordial greenhouse gases, higher bombard- ment rates, and higher volcanism; hence, the ‘‘?’’ associated with this negative feedback loop in Fig. 5 and Table 1. Without this abiotic feedback cycle to extend the outer edge of the CHZ, the much narrower Hart-like continuously habitable zone (Fig. 6B) becomes more plausible.
The other abiotic negative feedback on surface temperature shown in Fig. 5 is associated with low clouds: higher temper- atures / more volatilization / more low-altitude clouds / higher albedo / lower temperatures. Low clouds increase albedo and decrease surface temperatures more than they contribute toward raising the surface temperature because of the greenhouse effect associated with clouds (Abe et al., 2011). This is problematic because increasing volatilization produces both more low-altitude clouds, which could cool Earth due to their higher albedo, and more high-altitude clouds, which could
.
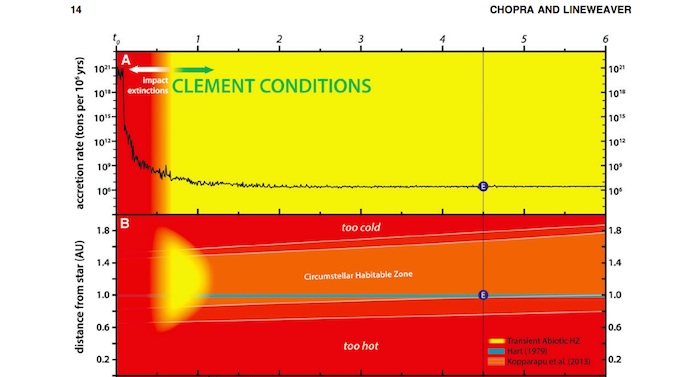
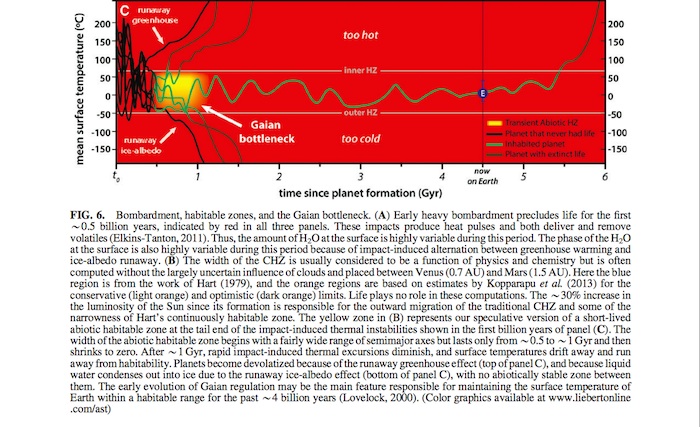
have a stronger greenhouse effect than albedo effect and thus increase surface temperatures (Goldblatt and Zahnle, 2011; Leconte et al., 2013). For this reason, the effects of clouds are often considered the biggest source of uncertainty in global climate models and thus the ‘‘?’’ associated with this cycle in Fig. 5 and Table 1.
While it may be possible to vary albedo and greenhouse gases within some plausible range and construct a wide CHZ, without negative feedback, there is no justification for tuning these abiotic variables to maintain habitability. We postulate that the abiotic stabilizing feedbacks (two cycles on the right side of Fig. 5) were probably negligible on early Earth. In their absence, it is hard to understand how habitability would have been maintained. Driverless cars do not stay on roads. Without significant abiotic stabilization, we propose that the most plausible default becomes the abiotic tendency to evolve away from habitability shown in Figs. 1 and 6C.
Just because Earth is at 1 AU and has been inhabited for *4 billion years does not mean that there is a physics-based, biology-independent, computable continuous habitable zone. With thermal instability and increasing stellar luminosity, it is not clear that a physics-based continuously habitable zone even exists. There may be no range of orbital distances (or any region of multidimensional abiotic parameter space) for which the surface environments of initially wet rocky planets have sufficiently strong abiotic negative feedback to maintain habitability. If this is the case, purely abiotic computations of a continuously habitable zone may be misleading, and Gaian regulation becomes a plausible explanation for the continu-
ously inhabited habitable zone in which we find ourselves.
5. The Biology of Habitability in the First Billion Years
It is usually assumed that the CHZ is determined by abiotic physical parameters: stellar mass and luminosity, planetary mass and atmospheric greenhouse gas composition, surface albedo, and sometimes clouds. More recently planetary spin, orbital eccentricity, obliquity, and initial water content have been added to the list of physical parameters (e.g., Gaidos et al., 2005; Gonzalez, 2005; Lammer et al., 2009; Gu ̈ del et al., 2014; Shields et al., 2016). Here, we argue that these abiotic pa- rameters can fleetingly enable the emergence of life but cannot maintain habitable surface conditions with liquid water. As the early heavy bombardment subsides, strong selection pressure on life begins to regulate, control, and even dominate the mechanisms that create or maintain the temperatures and pressures at the surface of a planet that allow liquid water. If so, then biology (rather than physics or chemistry) can play the most important role in maintaining habitability.
In addition to the abiotic environmental changes (due to bombardment and devolatilization), there could be a long struggle that starts early between life and an environment that does not, abiotically, stay habitable. Feedback between life and environment may play the dominant role in maintaining the habitability of the few rocky planets in which life has been able to evolve Gaian regulation quickly. If life gets started on a planet, there are many potential ways in which life can regulate the mechanisms that create or maintain the temperatures and pressures needed for liquid water (Schneider and Boston, 1991; Schneider, 2004; Harding and Margulis, 2010). Gaia researchers propose that life on Earth evolved to become integrated into previously abiotic feedback systems
that can modify or regulate surface temperature and the hydrological cycle (e.g., Lenton, 1998; Nisbet et al., 2012). Life can evolve to enhance and regulate the feedback loops (biological mediation processes A–D in Fig. 5 and Table 1). Biologically mediated feedback loops are stabilizing or Gaian (Ricklefs and Miller, 2000). For habitability to be maintained, life could down-regulate the positive runaway feedback loops and enhance the negative feedback loops. On Earth, life began to modulate the greenhouse gas com- position of the atmosphere as soon as life became wide- spread (Nisbet, 2002; Nisbet et al., 2012; Nisbet and Fowler, 2014; Johnson and Goldblatt, 2015).
The use of the Gaia hypothesis in ecology was reviewed by Free and Barton (2007). They argued [in agreement with Dawkins (1982)] that selection for global stability is implau- sible. However, they defined a Probable Homeostatic Gaia model: a planet ‘‘with appropriate starting conditions for life will probably generate a biosphere the lifespan of which will be extended, rather than reduced, by life-environment feed- back.’’ In their Probable Homeostatic Gaia model, a ‘‘network of life-environment interactions, largely dependent on the by- product effects of evolved traits, leads to global stability.’’ This biology-based global stability is what we call Gaian regulation. We are invoking its rapid evolution to stabilize the early volatile and thermal instabilities of wet rocky planets.
If Gaian regulation plays the dominant role in maintain- ing liquid water at the surface (Harding and Margulis, 2010), then the width of the CHZ would depend more on the quirks of biological evolution than on the more deterministic physics and chemistry that can be more easily modeled. For most rocky planets in the CHZ to remain habitable, they may have to be inhabited: ‘‘habitability depends on in- habitance and the width of the habitable zone is difficult to characterize’’ (Goldblatt, 2015).
In Gaian literature, it is usually proposed that Earth became a Gaian planet in the Proterozoic (*2.5 Gya) and has been one ever since (Harding and Margulis, 2010, p. 45). We are pro- posing that the onset of Gaian regulation could have occurred more than a billion years earlier, for example, through the production, consumption, and regulation of greenhouse active gases such as H2, CO2, and CH4. If there is a biotic solution for the faint early Sun paradox (Sagan and Mullen, 1972; Sagan and Chyba, 1997; Feulner, 2012) based on higher concentra- tions of CO2, on biotic methanogenesis, or on biotic albedo regulation, then this solution would necessarily have evolved quickly during the transient abiotic habitable zone (yellow regions in Fig. 6B, 6C) (Walker, 1985; Pavlov et al., 2003; Haqq-Misra et al., 2008; Rosing et al., 2010).
6. Predictions and Tests of the Gaian Bottleneck Hypothesis
After the early heavy bombardment, life emerges with some probability on initially wet rocky planets in the CHZ. However, due to large impacts and unstable abiotic volatile evolution with no tendency to maintain habitability, almost all life goes extinct early—with the rare exceptions of life that has undergone unusually rapid evolution and obtained some level of Gaian regulation. The most significant predictions of this Gaian bottleneck model can be seen in Fig. 7 by com- paring panels A and B with C. In panels A and B, wherever life emerges, it persists for billions of years. Thus, it has time
.
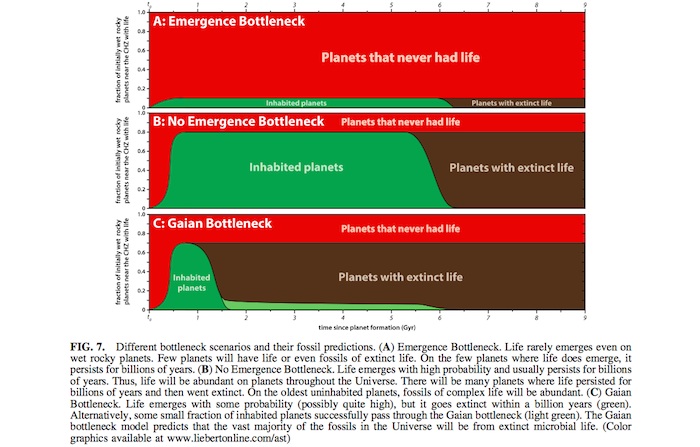
to evolve complex and perhaps multicellular forms. In panel C, which illustrates the Gaian bottleneck model, almost all emerging life goes extinct rapidly and therefore, does not have time to evolve into more complex forms. However, even planets with Gaian regulation will not be able to counter in- definitely the increasing luminosity due to stellar evolution (Caldeira and Kasting, 1992; Franck, 2000; Lenton and von Bloh, 2001; Franck et al., 2002; von Bloh et al., 2005). Hence, the extinction at *6 Gyr in all three panels.
If we are able to find well-preserved, *3.8 to *4.3 billion-year-old rocks on Venus or Mars, then we may be able to identify isotopic anomalies produced by biotic actions, in a way analogous to how 12C/13C ratios are used to infer the existence of the earliest life in Isua, Greenland (Ohtomo et al., 2014). Whether it evolved independently of life on Earth will be difficult to determine. If we find evidence of extant life on Mars or Venus that had an origin independent of Earth life, then this would be evidence against both the Gaian bottleneck hypothesis and the emergence bottleneck.
The surface temperature and existence of liquid water at, or near, the surface could be predominantly due to Gaian regulation rather than abiotic negative feedback. Liquid water on the surface of the planet (particularly old planets) would then not just be a prerequisite for life but a bio- signature (Gorshkov et al., 2004). Existence of liquid water
on the surface of a planet may be a better biosignature than oxygen (Luger and Barnes, 2015). Thus, the measurement of exoplanetary surface temperatures compatible with liquid water could be an important part of future search for ex- traterrestrial life. Remote detection of atmospheric chemical equilibrium may soon develop into a mature science of remote biodetection (e.g., Lovelock and Kaplan, 1975; Krissansen-Totton et al., 2016). The Gaian bottleneck model predicts that the vast majority of the atmospheres of old terrestrial planets in the traditional abiotic CHZs of their host stars will be in chemical equilibrium because they are uninhabited. Hence, atmospheres in chemical disequilibrium will be rare except for young (t ( 2 Gyr) terrestrial planets.
In a critique of Gaian logic, Dawkins (1982, p. 236) wrote:
For the analogy [of the Earth as an organism] to apply strictly, there would have to have been a set of rival Gaias, presumably on different planets. Biospheres which did not develop efficient homeostatic regulation of their planetary atmospheres tended to go extinct. The Universe would have to be full of dead planets whose homeostatic regulation systems had failed, with, dotted around, a handful of suc- cessful, well-regulated planets of which the Earth is one.
What Dawkins describes here is also a prediction of the Gaian bottleneck hypothesis. The Gaian bottleneck model parallels evolution on Earth in that the vast majority (*99.9%) of species that have ever lived are now extinct; the vast majority of planetary life has gone extinct.
In the far future, we may be able to find evidence for biogenic isotopic anomalies on the initially wet rocky planets around most stars. Since life does not persist for long in the Gaian bottleneck model, it predicts a universe filled with isotopic or microscopic fossils from the kind of life that can evolve in *1 billion years, not the fossils of larger multicellular eukaryotes or anything else that would take several billion years to evolve.
Cockell (2014) divided all environments in the Universe into three types: (1) uninhabitable, (2) uninhabited habitats, or (3) inhabited habitats (Cockell, 2011; Zuluaga et al., 2014). A prediction of the Gaian bottleneck (persistence-is- hard) model is that (2) and (3) will be rare. This is unfor- tunate for future colonization efforts since uninhabited, but habitable, planets are the most ethically appealing places— autochthonous life would not have to be displaced.
Our search for life beyond Earth may be thwarted by the short timescales over which planets may remain inhabited. If it takes several billion years to develop radio telescopes, then the Gaian bottleneck ensures that the vast majority of life in the Universe is either young and microbial, or extinct. Therefore, the Gaian bottleneck model is consistent with current SETI results and can help resolve Fermi’s paradox, although it is not one of the solutions to the paradox listed by Webb (2002).
7. What Could Be Wrong with Our Argument?
(1) Gaian regulation is a controversial idea. It is usually invoked to explain the long-term stability of the surface temperature of Earth, starting in the Protero- zoic (*2.5 Gya). So invoking early, pre-Proterozoic Gaia is even more controversial.
(2) If estimates of sub-aerial continental crust (e.g., by Flament et al., 2008) are significantly too low and there was abundant sub-aerial crust earlier than *3.8 Gya (e.g., Van Kranendonk, 2010), then abiotic negative feedback based on the carbonate-silicate cycle could have stabilized surface temperatures very early in Earth’s history, without Gaian regulation. If early continent formation is a common feature of rocky planets, then invoking Gaian regulation may be unnecessary to explain Earth’s early thermal stability.
(3) We are arguing that
(a) Gaian regulation evolved on Earth.
(b) The evolution of Gaian regulation is not common.
This could seem paradoxical, because to justify (a) we have presented arguments making the emergence of Gaian regulation plausible. These same arguments could suggest that Gaian regulation would be com- mon. However, there is a large class of phenomena that did happen on Earth that are uncommon or nonexistent elsewhere. We can trace the evolution of these phenomena and explain how they evolved on Earth, but these explanations cannot be generalized. For example, the evolution of the English language can be traced and understood and made plausible, but this plausibility cannot be turned into a generic ar- gument for the evolution of English on other planets.
Humanlike intelligence may be another member of this class (Lineweaver, 2008). We are suggesting that the evolution of a kind of life that can quickly and effectively regulate the volatiles on the surface of the planet on which it finds itself may be a quirky product of the biological evolution of life on early Earth.
(4) Why should Gaian regulation be rare? Franck et al. (2002, 2001) concluded that there are *500,000 ‘‘sister Gaias’’ in the Milky Way Galaxy but then listed a number of physical factors [e.g., those discussed by Ward and Brownlee (2000)] that could reduce this estimate.
(5) It could be that early heavy impacts almost always extinguish life and do not need any help from subse- quent volatile evolution away from habitability. In which case, Gaian regulation would have nothing to do with life’s early or late extinction. Evidence for this would be an anomalously low impact rate on Earth (compared to the early impact rates on other wet rocky planets). If this were the case, there would be no ten- dency for life to evolve and regulate its environment. In this context, Tyrrell (2013) suggested that ‘‘Lucky Gaia’’ as described by Free and Barton (2007) and the anthropic principle provide a better explanation for the continued habitability of Earth than ‘‘Probable Gaia,’’ which we assume here.
If the probability of the emergence and persis- tence of life on wet rocky planets were infinitesi- mally small and there were only one life-harboring planet in the Universe, we would, of necessity, find ourselves on that planet. Therefore, our mere exis- tence cannot be used to infer the probability of life elsewhere. Additionally, invoking a scenario in which persistent life on Earth is exceptional com- pared to other planets (as we do here) cannot be criticized with an argument such as, if Gaian regu- lation is rare, then we should not be here. Self- selection overcomes this critique.
(6) One argument against early Gaian regulation is that the unit of biological selection starts small and moves to larger groups as in the following chronological sequence: genes, chromosomes, single cells, colonies of cells (bacterial mats), multicellular organisms, colonies of multicellular organisms (superorganisms), ecosystems of various sizes (which produce Gaian regulation only when they are widespread). In this sequence, the evolution of Gaian regulation happens last. However, among the earliest life-forms we know of, stromatolites were already ecosystems of bacterial mats (Walter et al., 1992).
(7) The Universe does not seem to be teeming with life. This could be an observational selection effect: it is teeming with life, but we just have not been able to detect it yet. Even in the future when the remote detection of biosignatures is possible, it will be dif- ficult to detect subsurface life (Boston et al., 1992; Gaidos et al., 1999; Jones et al., 2011; McMahon et al., 2013) that does not interact with the planet’s atmosphere and is completely sustained by free en- ergy based on geochemical disequilibrium. This would undermine some of the motivation for the Gaian bottleneck model but not the other arguments presented here.
8. Conclusion
We are proposing a potentially universal sequence of events on initially wet rocky planets that can be summarized thusly:
First *0.5 Gyr: Hot, high bombardment, uninhabitable.
*0.5 to *1.0 Gyr: Cooler, reduced bombardment, con- tinuous volatile loss.
*0.5 to *1.0 Gyr: Emergence of life in an environment with a tendency to evolve away from habitability.
*1.0 to *1.5Gyr: Inability to maintain habitability, followed by extinction. As a rare alternative, this period would experience the rapid evolution of Gaian regulation and the maintenance of habitability, followed by the per- sistence of life for several billion more years.
Between the early heat pulses, freezing, volatile content variation, and runaway positive feedbacks, maintaining life on an initially wet rocky planet in the habitable zone may be like trying to ride a wild bull. Most life falls off. Life may be rare in the Universe, not because it is difficult to get started, but because habitable environments are difficult to maintain during the first billion years.
In the book Vital Dust, de Duve (1995) presented the case that water and energy are common and abiogenesis may be a cosmic imperative. The most important constraint on the existence of life in the Universe may be whether life, after emerging and evolving into a biosphere, can evolve global mechanisms rapidly enough to mediate the positive and negative feedbacks of abiotic atmospheric evolution. We hypothesize that the early evolution of biologically mediated negative feedback processes, or Gaian regulation as pro- posed by Lovelock and Margulis (1974), may be necessary to maintain habitability because of the strength, rapidity, and universality of abiotic positive feedbacks on the sur- faces of rocky planets in traditional CHZs.
We argue that the habitable surface environments of rocky planets usually become uninhabitable due to abiotic runaway positive feedback mechanisms involving surface temperature, albedo, and the loss of atmospheric volatiles. Because of the strength, rapidity, and universality of abiotic positive feedbacks in the atmospheres of rocky planets in traditional CHZs, biotic negative feedback or Gaian regu- lation may be necessary to maintain habitability.
The evolution of biospheric regulation of surface vola- tiles, temperature, and albedo can become a Gaian bottle- neck to the persistence of life. This Gaian bottleneck may be a better explanation for the nonprevalence of life than the traditional emergence bottleneck paradigm.
Acknowledgments
A.C. acknowledges PhD scholarship funding from the Australian Postgraduate Award and the Research School of Earth Sciences at the Australian National University. We thank Jim Kasting, David Catling, and Vickie Bennett for helpful discussions. We thank Norm Sleep and an anony- mous reviewer for their insightful feedback and constructive suggestions.
Quelle:
ASTROBIOLOGY
Volume 16, Number 1, 2016
4034 Views