.
18.09.2015
COMET SURFACE CHANGES BEFORE ROSETTA’S EYES
In the months leading to the perihelion of Comet 67P/Churyumov-Gerasimenko, Rosetta scientists have been witnessing dramatic and rapid surface changes on the Imhotep region, as reported in a paper to be published in Astronomy & Astrophysics
Since arriving at Comet 67P/C-G in August 2014, Rosetta has been witnessing an increase in the activity of the comet, warmed by the ever-closer Sun. A general increase in the outflow of gas and dust has been punctuated by the emergence of jets and dramatic rapid outbursts in the weeks around perihelion, the closest point to the Sun on the comet’s orbit, which occurred on 13 August 2015.
But in June 2015, just two months before perihelion, Rosetta scientists started noticing important changes on the surface of the nucleus itself. These very significant alterations have been seen in Imhotep, a region containing smooth terrains covered by fine-grained material as well as large boulders, located on 67P/C-G’s large lobe.
.
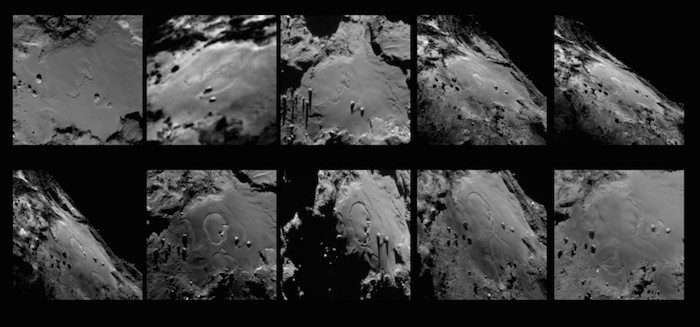
Sequence of ten images showing changes in the Imhotep region on Comet 67P/C-G. The images were taken with the OSIRIS narrow-angle camera on Rosetta between 24 May and 11 July 2015. The individual images are also available separately. Credits: ESA/Rosetta/MPS for OSIRIS Team MPS/UPD/LAM/IAA/SSO/INTA/UPM/DASP/IDA
-
“We had been closely monitoring the Imhotep region since August 2014, and as late as May 2015, we had detected no changes down to scales of a tenth of a metre,” comments Olivier Groussin, an astronomer at the Laboratoire d'Astrophysique de Marseille, France, OSIRIS Co-Investigator and lead author of the study.
“Then one morning we noticed that something new had happened: the surface of Imhotep had started to change dramatically. The changes kept going on for quite a while.”
First evidence for a new, roughly round feature in Imhotep was seen in an image taken with Rosetta’s OSIRIS narrow-angle camera on 3 June. Subsequent images later in June showed this feature growing in size, and being joined by a second round feature. By 2 July, they had reached diameters of roughly 220 m and 140 m, respectively, and another new feature began to appear.
By the time of the last image used in this study, taken on 11 July, these three features had merged into one larger region and yet another two features had appeared.
.
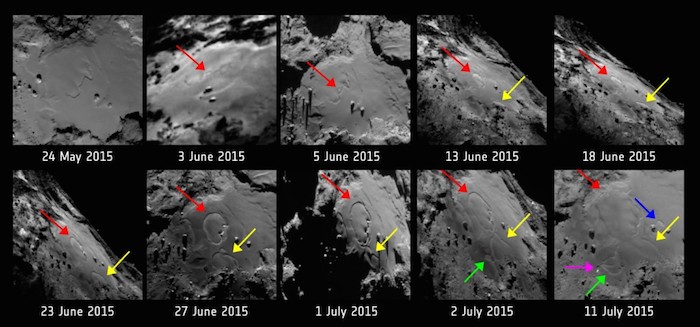
Same sequence as above, with indication of dates and location of the morphological changes. Credits: ESA/Rosetta/MPS for OSIRIS Team MPS/UPD/LAM/IAA/SSO/INTA/UPM/DASP/IDA
-
“These spectacular changes are proceeding extremely rapidly, with the rims of the features expanding by a few tens of centimetres per hour. This highlights the complexity of the physical processes involved,” adds Olivier.
The sublimation of volatile species is clearly an important factor, as colour images of this region reveal the signature of exposed ice on some of the rims of the newly-formed surface features. The rapid rate of expansion is unexpected, however: models of sunlight-driven sublimation would predict erosion rates of just a few centimetres per hour, and thus the scientists believe that additional mechanisms are required to explain the observations.
A simple possibility is that the surface material is very weak, allowing for more rapid erosion, but it is also possible that the crystallisation of amorphous ice or the destabilisation of so-called ‘clathrates’ (a lattice of one kind of molecule containing other molecules) could liberate energy and thus drive the expansion of the features at faster speeds.
.
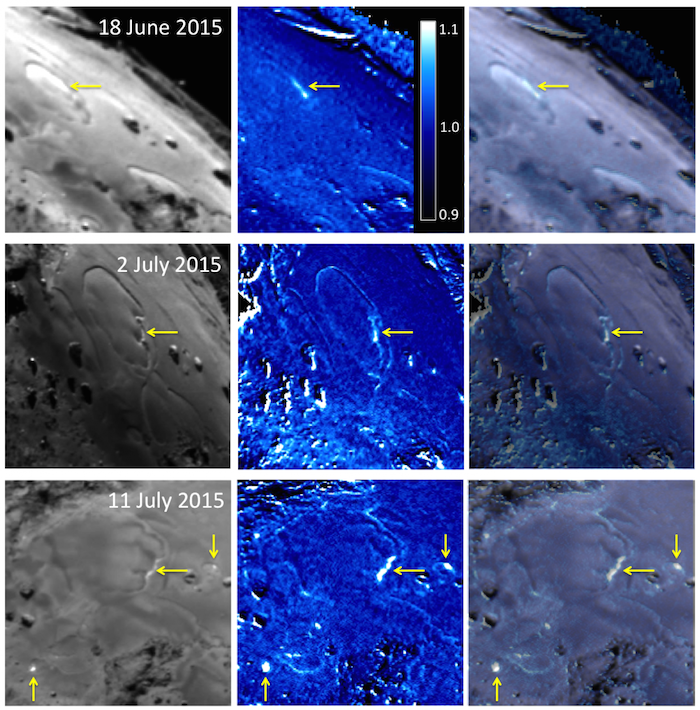
Colour images of the Imhotep region on Comet 67P/C-G, taken with the OSIRIS narrow-angle camera on Rosetta on 18 June (upper row), 2 July (middle row) and 11 July 2015 (lower row). The first column shows images taken in the orange filter (649 nanometres); the second column shows the ratio between images taken with the blue filter (481 nanometres) and the orange filter for the 18 June and 2 July images, and the ratio between images taken with the blue and the red (701 nanometres) filters for the 11 July image; the third column shows a composite obtained by combining the images in the previous two columns. The yellow arrows indicate some of the new features that were detected on Imhotep. These colour images show that some patches on the surface of the comet reflect orange/red light less effectively and blue light more effectively than their surroundings. They appear as white in the central column, where the colour ratio is shown. This indicates the presence of frozen water ice at or just below the surface of these patches. Credits: ESA/Rosetta/MPS for OSIRIS Team MPS/UPD/LAM/IAA/SSO/INTA/UPM/DASP/IDA
-
The erosion could be accompanied by increased rates of gas outflow, including H2O, CO2, or CO. The scientists also searched in OSIRIS images for evidence of increased dust rising from Imhotep as the surface morphology evolved, but did not find any.
While it is unlikely that many small (micron-sized) dust particles were released as the features formed and expanded, it is possible that the same amount of mass was released in a smaller number of larger (millimetre-sized) particles, which would produce less reflected light and thus be harder to detect with OSIRIS.
In addition, a significant fraction of the dust released may have immediately fallen back to the surface, accumulating at the base of the expanding rims.
.
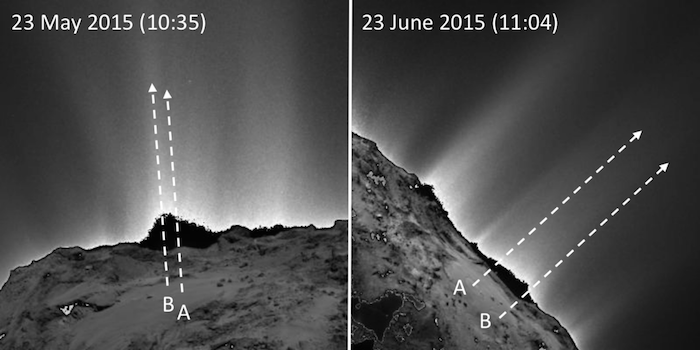
Activity seen above the Imhotep region with the OSIRIS narrow-angle camera on Rosetta on 23 May 2015 (left), before significant morphological changes were seen in this region, and on 23 June 2015 (right), after the changes had begun to appear. (Times are in UT.) The positions of the first two new features that were seen in Imhotep are marked with A and B. The white arrows indicate the direction along which an increase of activity would have been seen in the case of jets lifting from the newly arisen features. Credits: ESA/Rosetta/MPS for OSIRIS Team MPS/UPD/LAM/IAA/SSO/INTA/UPM/DASP/IDA
Although the scientists were initially surprised to see such significant changes taking place on smooth terrains such as those seen in Imhotep, the location of this region close to the comet’s equator guarantees that it receives large amounts of sunlight.
“We are looking forward to combining our OSIRIS observations with data from other instruments on Rosetta, to piece together the origin of these curious features,” concludes Olivier.
Quelle: ESA
---
ROSETTA’S FAR EXCURSION TO STUDY THE COMA AT LARGE
Next week, on 23 September, Rosetta will depart on a three-week excursion that will take it up to 1500 km from the nucleus of Comet 67P/Churyumov-Gerasimenko, much farther than it has been since arriving at the comet in August 2014.
.
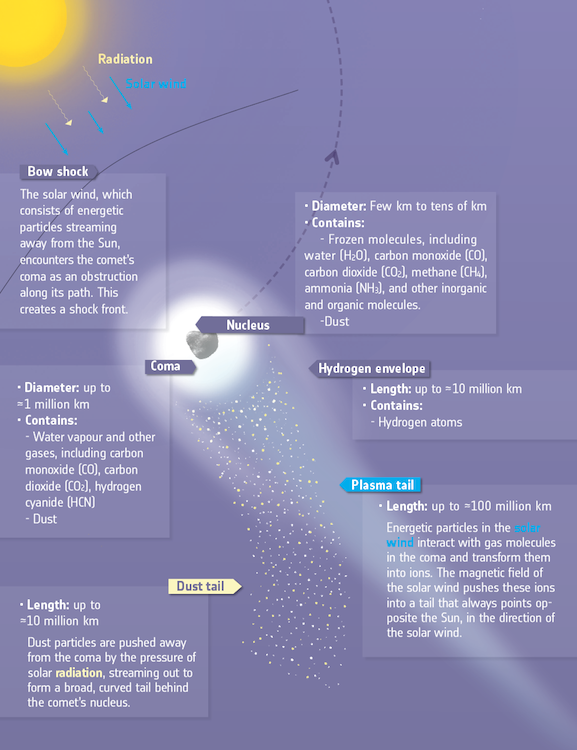
The various components of a comet, including the bow shock. Credit: ESA
.
The main science goal driving this course of action is to study the coma of 67P/C-G on a broader scale while the comet's activity is still high in the post-perihelion phase. While almost all instruments on Rosetta will be operating during the excursion, this exploration of the coma at large will be especially interesting to study the plasma environment of the comet with the Rosetta Plasma Consortium (RPC) instruments.
In particular, scientists are aiming at detecting the bow shock, a boundary between the comet's magnetosphere and the ambient solar wind. The existence of a bow shock in a comet's environment around its activity peak was predicted in 1967 by Ludwig Biermann, and confirmed in the past decades by observations at comets 21P/Giacobini–Zinner, 1P/Halley, 26P/Grigg–Skjellerup and 19P/Borrelly.
“Previous measurements that were performed during fly-bys only provided limited data points about the bow shocks of a handful of comets. Rosetta, instead, will take data over several days, monitoring the evolution of the plasma environment of 67P/C-G shortly after its perihelion,” says Claire Vallat, a Rosetta Science Ground Segment scientist at ESA's European Space Astronomy Centre (ESAC).
Along the new trajectory, Rosetta will move away from the nucleus up to 1500 km in the direction of the Sun, where the bow shock is expected to be found. This maximum distance will be reached by the end of September, with the spacecraft returning to closer distances by mid-October.
.
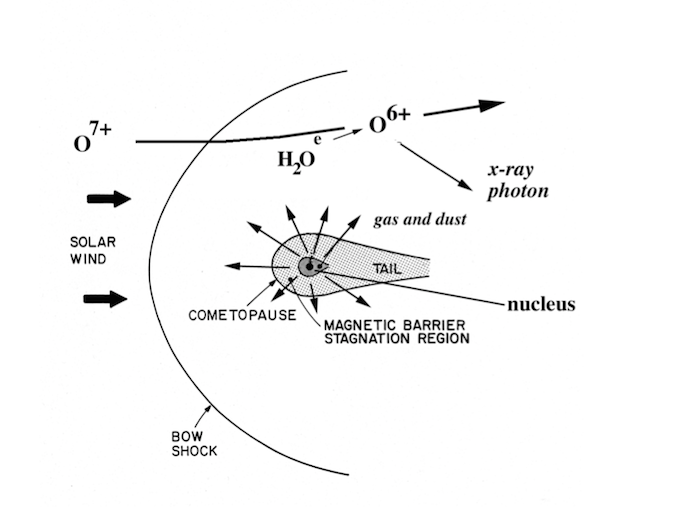
The plasma environment of an active comet. From T. E. Cravens & T. I. Gombosi, Cometary Magnetospheres: a tutorial, 2004, Advances in Space Research, Volume 33, Issue 11, p. 1968-1976
.
“While it may appear odd to depart from the nucleus at this time, these measurements are also key to understanding the comet's behaviour at large and must be performed not too long after perihelion, so that the comet is still appreciably active,” adds Claire.
Departure on the 1500-km excursion will be kicked off by an early morning thruster burn set for 01:40 GMT (03:40 CEST) on 23 September. The burn commands for a 2.34 m/s impulse push will be uploaded in advance and Rosetta will be pushed onto a slow escape path.
After the burn is complete, Rosetta will move out from its current orbit, approximately 450 km from the nucleus, aiming at the farthest point on the excursion with a phase angle of 50 deg, and arriving at 1500 km from the comet on 30 September. On that date, the spacecraft will be arriving on the morning side of 67P/C-G, over the comet's southern hemisphere, at -60 degrees latitude.
“Once we're far from the comet, we won't be able to identify landmarks for navigation anymore as we'll be too far out. Navigation will be based on the determination of the comet centre in NavCam images,” says Spacecraft Operations Manager Sylvain Lodiot at ESA's European Space Operations Centre (ESOC).
Having reached the farthest point on this stretch, Rosetta will conduct a return burn, which will bring it back to about 500 km above the comet by 7 October. While the spacecraft is on the excursion, the cometary environment will continue to evolve, but the mission operations team won't have a firm, up-to-date characterisation of the level of activity, so will make the return approach cautiously.
“We won't stay at 500 km, but we'll only get closer step by step, as we understand what's then happening at the comet and regain knowledge of its activity,” says Lodiot.
As a comet gets closer to the Sun, frozen molecules – including water, carbon monoxide and carbon dioxide – both on and below the nucleus surface sublimate. As the outflowing gases leave the nucleus, they carry dust particles along and, together, they produce the comet's coma.
.
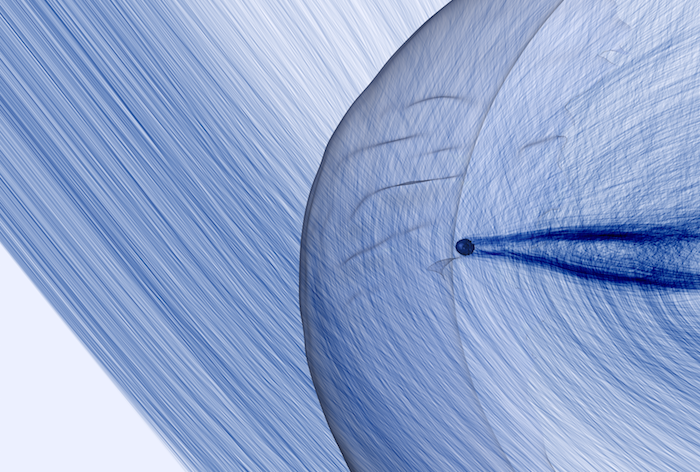
Visualisation of the magnetic field lines in the comet plasma environment. The “undisturbed” interplanetary magnetic field is visible on the left, the bow shock at the centre and the magnetic field draped around the comet on the right. The small blue sphere, with a radius of about 100 km, shows the size of the innermost coma, which contains the diamagnetic cavity, the ion and magnetic field pile-up regions. Credits: Modelling and simulation: Technische Universität Braunschweig and Deutsches Zentrum für Luft- und Raumfahrt; Visualisation: Zuse-Institut Berlin
.
The molecules in the coma are originally neutral, but can be stripped off of one or more of their electrons, thus becoming ionised, due to a variety of physical processes in the comet's environment. The resulting molecular ions, such as H2O+ and O+, build up the comet's magnetosphere and start interacting with the solar wind – a stream of charged particles and ions flowing from the Sun throughout the solar system.
The cometary ions, which move very slowly with respect to the high-speed flow of the solar wind, are “picked up” by the solar wind, adding more and more mass to its flow. As a consequence, the solar wind feels the presence of an obstacle, represented by the active comet, and decelerates gradually, until eventually a discontinuity arises with a sharp difference of the magnetic field values between the two plasma environments: the bow shock.
During fly-bys of previously visited comets, bow shocks were detected at distances of several thousands of km from the nucleus. In 1986, ESA's Giotto mission measured a bow shock around one million km away from the nucleus of comet 1P/Halley; later, in 1992, it detected another bow shock during its fly-by of comet 26P/Grigg–Skjellerup, about 20,000 km from the nucleus.
.
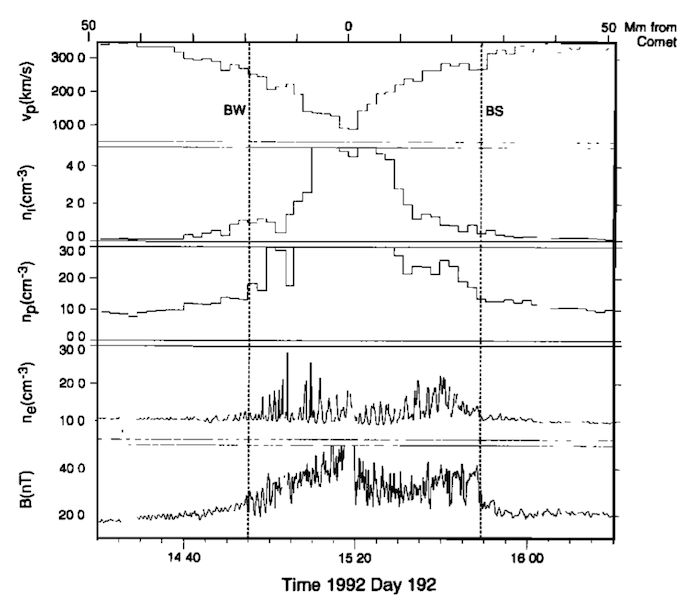
The plasma parameters measured by ESA's Giotto mission in 1992, during its fly-by of Comet 26P/Grigg–Skjellerup. From A. J. Coates et al, 1997, Journal of Geophysical Research, vol. 102, no. A4, pages 7105.
.
“The location of the bow shock depends on the comet's activity,” explains Hans Nilsson from the Swedish Institute for Space Physics, who is the Principal Investigator of the Ion Composition Analyser – one of the RPC instruments.
“Comet 1P/Halley was much more active than 67P/C-G, and the bow shock was much further away than what we expect to find with Rosetta. On the other hand, 26P/Grigg-Skjellerup was a relatively low-activity comet, and its gas production rate at the time of the Giotto encounter was similar to that of 67P/C-G at the time of perihelion.”
While Rosetta will not venture this far from the nucleus, the timing of the far excursion – six to eight weeks after perihelion – was planned in such a way that the bow shock will be closer to the nucleus.
“Hybrid plasma simulations indicate that a bow shock should have formed by now, and that we should see it around a thousand km from the nucleus,” explains Christoph Koenders, an RPC scientist from the Institute for Geophysics and Extraterrestrial Physics at the Technische Universität Braunschweig, Germany.
“The exact location of the boundary depends on the solar wind velocity and density, on the comet's gas production rate and on the interplanetary magnetic field, and small variations in these parameters might shift it considerably. However, we are confident that we will detect the bow shock at some point during the excursion.”
.
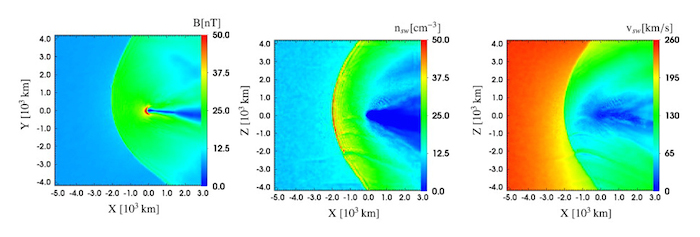
Visualisations from a hybrid plasma simulation of the interaction of Comet 67P/Churyumov–Gerasimenko at a distance of 1.3 Astronomical Units from the Sun, showing the strength of the magnetic field in the z=0 plane (left frame), the density (middle frame) and the velocity (right frame) of the solar wind protons. Adapted from C. Koenders et al, 2013, Planetary and Space Science, Vol. 87, Pages 85–95.
-
During the far excursion starting on 23 September, RPC scientists are planning to sample the magnetosphere of 67P/C-G at a range of distances from the nucleus that have not been probed yet, measuring the properties of ions and electrons and the magnetic field in the plasma environment. Besides the bow shock, they expect to detect several other transition regions, such as a cometopause and a cometary magnetosheath, as well as some other possible boundaries, which will all show a unique signature in each of the measurements.
While the temporal resolution of the data will be similar to that obtained during previous fly-bys of other comets, the spatial resolution will be improved by several orders of magnitude thanks to Rosetta's much lower velocity with respect to the comet. In addition, there will be a chance to study temporal variations of the comet's plasma environment, as the spacecraft will spend significant time in each region of the comet magnetosphere.
“Shocks are a ubiquitous phenomena in astrophysics and studying them in situ is a great way to get at the physics,” says Matt Taylor, Rosetta project scientist at ESA.
“For example, ESA's Cluster mission explored the remarkably thin bow shock of our own planet a few years ago, revealing that it is an ideal site for particle acceleration. Now, Rosetta will allow us to study a bow shock of a very different celestial body in great detail. Since the conditions at this comet are just on the limit for the bow shock to form, we will have a chance to investigate in great detail how these boundaries arise.”
Scientists are looking forward to using these data to learn about the formation of shock waves and other boundaries in the plasma environment of a comet, and to examine how these affect the transfer of energy and momentum from the solar wind to the comet's atmosphere. Comet 67P/C-G provides a new environment that allows to study the interaction of the solar wind in a context that is much different from that of a planet.
Quelle: ESA
-
Update: 24.09.2015
.
The water-ice cycle of Rosetta’s comet
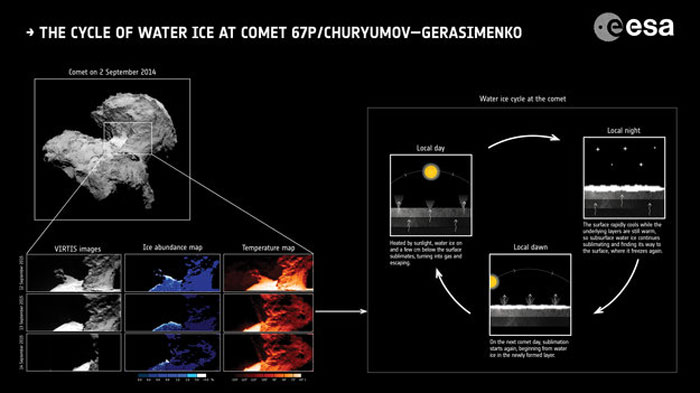
ESA’s Rosetta spacecraft has provided evidence for a daily water-ice cycle on and near the surface of comets.
Comets are celestial bodies comprising a mixture of dust and ices, which they periodically shed as they swing towards their closest point to the Sun along their highly eccentric orbits.
As sunlight heats the frozen nucleus of a comet, the ice in it – mainly water but also other ‘volatiles’ such as carbon monoxide and carbon dioxide – turns directly into a gas.
This gas flows away from the comet, carrying dust particles along. Together, gas and dust build up the bright halo and tails that are characteristic of comets.
Rosetta arrived at Comet 67P/Churyumov–Gerasimenko in August 2014 and has been studying it up close for over a year. On 13 August 2015, the comet reached the closest point to the Sun along its 6.5-year orbit, and is now moving back towards the outer Solar System.
A key feature that Rosetta’s scientists are investigating is the way in which activity on the comet and the associated outgassing are driven, by monitoring the increasing activity on and around the comet since Rosetta’s arrival.
Scientists using Rosetta’s Visible, InfraRed and Thermal Imaging Spectrometer, VIRTIS, have identified a region on the comet’s surface where water ice appears and disappears in sync with its rotation period. Their findings are published today in the journal Nature.
“We found a mechanism that replenishes the surface of the comet with fresh ice at every rotation: this keeps the comet ‘alive’,” says Maria Cristina De Sanctis from INAF-IAPS in Rome, Italy, lead author of the study.
.
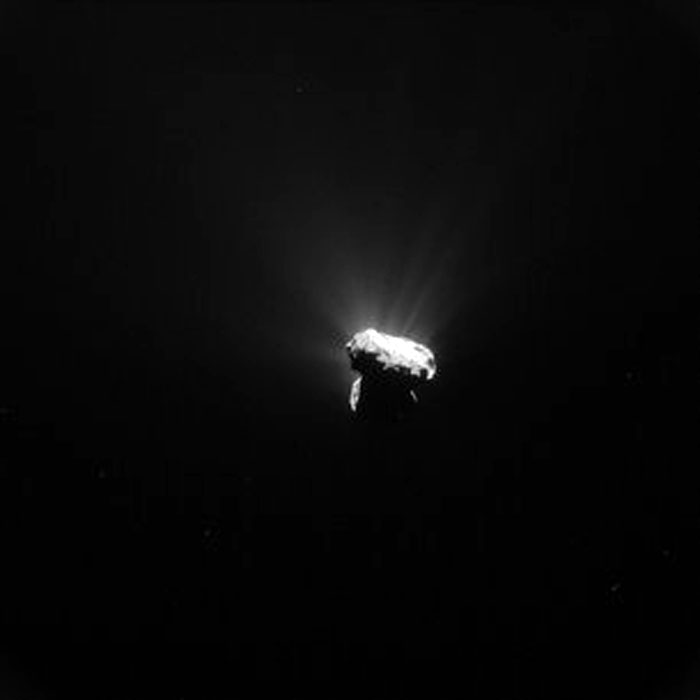
Comet at perihelion
.
The team studied a set of data taken in September 2014, concentrating on a one square km region on the comet’s neck. At the time, the comet was about 500 million km from the Sun and the neck was one of the most active areas.
As the comet rotates, taking just over 12 hours to complete a full revolution, the various regions undergo different illumination.
“We saw the tell-tale signature of water ice in the spectra of the study region but only when certain portions were cast in shadow,” says Maria Cristina.
“Conversely, when the Sun was shining on these regions, the ice was gone. This indicates a cyclical behaviour of water ice during each comet rotation.”
The data suggest that water ice on and a few centimetres below the surface ‘sublimates’ when illuminated by sunlight, turning it into gas that then flows away from the comet. Then, as the comet rotates and the same region falls into darkness, the surface rapidly cools again.
.
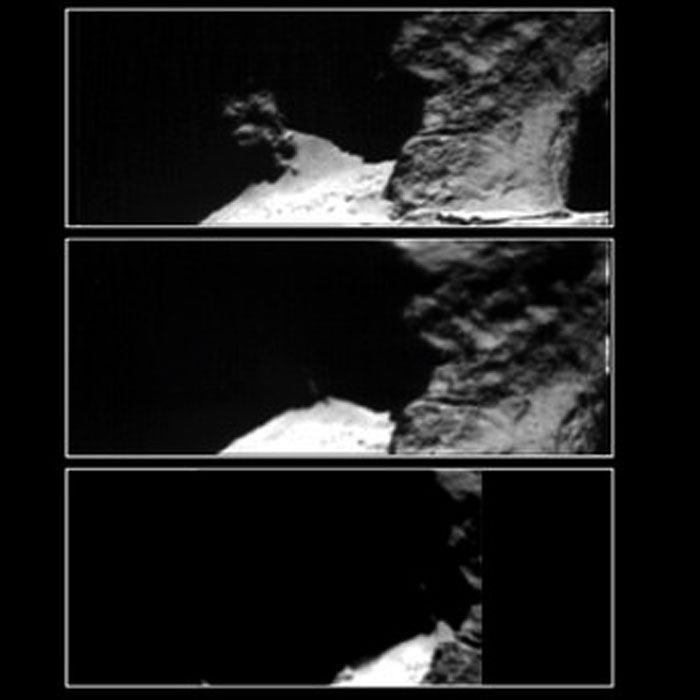
Hapi region
.
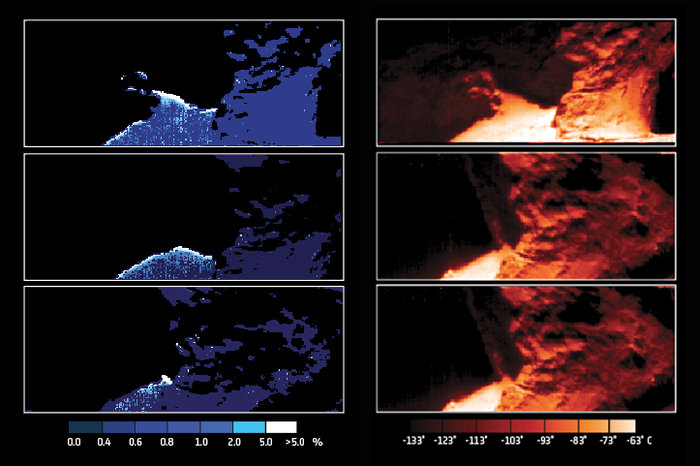
Title Water ice and surface temperature at Hapi
Released 23/09/2015 7:00 pm
Copyright ESA/Rosetta/VIRTIS/INAF-IAPS/OBS DE PARIS-LESIA/DLR; M.C. De Sanctis et al (2015)
Description
Maps of water ice abundance (left) and surface temperature (right) focusing on the Hapi ‘neck’ region of Comet 67P/Churyumov–Gerasimenko. These maps are based on images and spectra collected with Rosetta’s Visible, InfraRed and Thermal Imaging Spectrometer, VIRTIS on 12 (top), 13 (middle) and 14 September (bottom) 2014.
The ice abundance map is based on images taken with VIRTIS in the optical band at 0.7 micron and VIRTIS infrared spectra, combined with models of the surface and near-surface material. The infrared spectra taken in low illumination conditions show a strong dip at wavelengths between 2.7 and 3.6 micron, showing the characteristic shape of an absorption feature caused by water ice on the surface. In the water ice abundance maps, white indicates higher abundance of ice on the surface (over 5%) while blue hues indicate lower abundances: the darker blue, the lower abundance of ice, down to 0%.
The surface temperature maps are based on VIRTIS spectra at wavelengths above 4.5 micron. White and brighter hues indicate higher temperatures, with the highest temperature shown reaching –63ºC; darker and redder hues indicate lower temperatures, with the darkest hues indicating surface temperatures around –133ºC.
The 12 and 13 September maps are separated by about one comet rotation, while the 13 and 14 September maps are separated by three rotations. Due to the complex topography of the comet, the illumination conditions were different in each of the three occasions.
By comparing the two series of maps, the scientists have found that, especially on the left side of each frame, water ice is more abundant on colder patches (white areas in the water ice abundance maps, corresponding to darker areas in the surface temperature maps), while it is less abundant or absent on warmer patches (dark blue areas in the water ice abundance maps, corresponding to brighter areas in the surface temperature maps). In addition, water ice was only detected on patches of the surface when they were cast in shadow.
This indicates a cyclical behaviour of water ice during each comet rotation.
Quelle: ESA
-
Update: 25.09.2015
.
COMETWATCH 21 SEPTEMBER
Today's CometWatch entry was taken by Rosetta’s NAVCAM on 21 September 2015, about 330 km from the nucleus of Comet 67P/Churyumov-Gerasimenko.
.
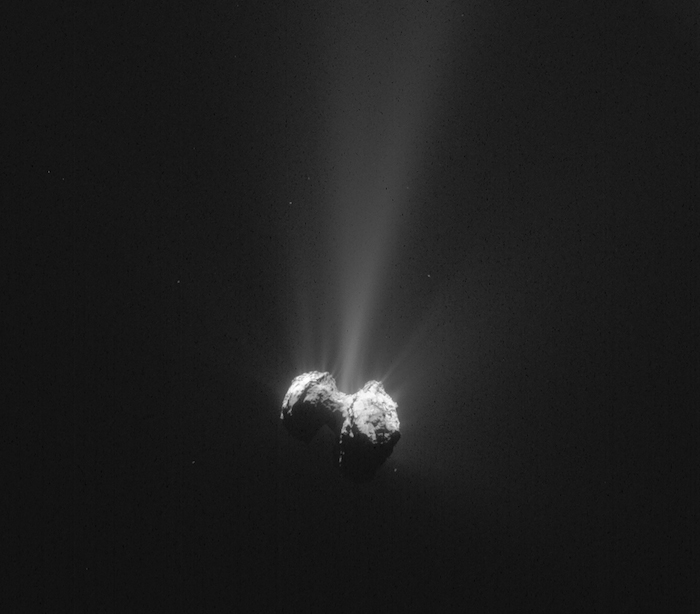
Single frame enhanced NAVCAM image of Comet 67P/C-G taken on 21 September 2015. Credits: ESA/Rosetta/NAVCAM – CC BY-SA IGO 3.0
.
The scale is 28.1 m/pixel and the image measures 28.8 km across. The contrast was increased to enhance the comet's activity; the original image is provided below.
The comet is oriented with the small lobe to the left and the large lobe to the right. Jets of gas and dust are seen all around the sunlit portion of the nucleus and are particularly clear around the central neck region with the ejected material seen extending towards the edge of the image frame.
This week Rosetta embarked on a new trajectory that will take it 1500 km away from the comet nucleus by 30 September in order to study the broader scale of the coma and to investigate the comet’s plasma environment. It will return to closer distances in mid-October.
---
ROSINA DETECTS ARGON AT COMET 67P/C-G
The noble gas argon has been detected in the coma of Comet 67P/Churyumov-Gerasimenko for the first time, thanks to the ROSINA mass spectrometer on-board Rosetta. Its detection is helping scientists to understand the processes at work during the comet’s formation, and adds to the debate about the role of comets in delivering various ‘ingredients’ to Earth.
The new results are reported in Science Advances today and describe data collected on 19, 20, 22, and 23 October 2014, when the comet was around 465 million km (3.1 AU) from the Sun, and Rosetta was in a 10 km orbit around the comet.
During the time spent close to the comet, the ROSINA instrument was able to take an inventory of the key constituents of the comet’s coma, with many ingredients already reported (see links at end of article). Determining the chemical make-up of comets is a necessary step to understanding their role in bringing water and other ingredients to the inner planets during the Solar System’s early history.
The so-called noble gases (helium, neon, argon, krypton, xenon, and radon) rarely react chemically with other elements to form molecules, mostly remaining in a stable atomic state, representative of the environment around a young star in which planets, comets, and asteroids are born.
.
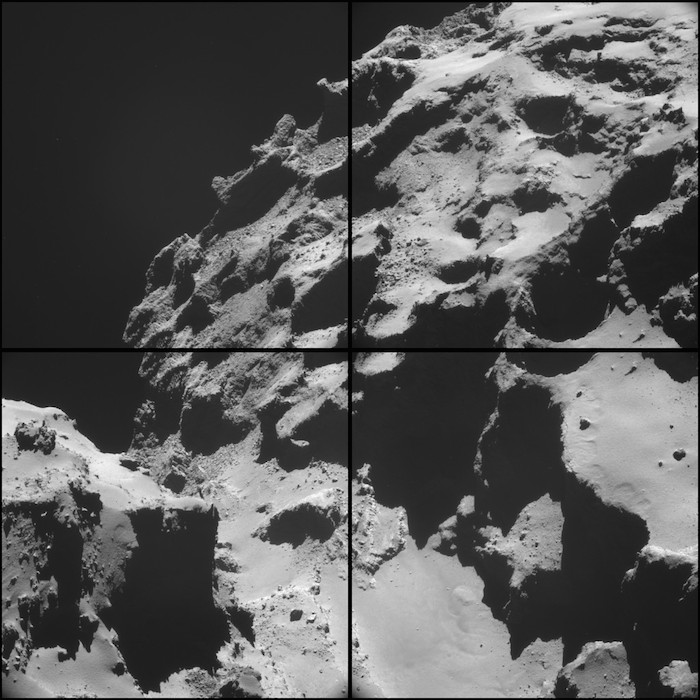
Four image NAVCAM montage of Comet 67P/C-G comprising images taken on 20 October 2014, during the timeframe of the ROSINA measurements reported today. The images were taken about 7.4 km from the comet surface. Credits: ESA/Rosetta/NAVCAM
.
In addition, their abundance and isotopic compositions can be compared to the values known for Earth and Mars, and for the solar wind and meteorites, for example. The relative abundance of noble gases in the atmospheres of terrestrial planets is largely controlled by the early evolution of the planets, including outgassing via geological processes, atmospheric loss, and/or delivery by asteroid or cometary bombardment. Thus the study of noble gases in comets can also provide information on these processes.
However, noble gases are very easily lost from comets through sublimation, and so this first detection of argon at Comet 67P/C-G is a key discovery. Not only that, but it is also an important step in determining if comets of this type played any significant role in the noble gas inventory of the terrestrial planets.
Scientists analysing data from ROSINA’s high-resolution Double Focusing Mass Spectrometer (DFMS) identified argon, along with other gases, in the coma spectra of Comet 67P/C-G in October 2014. They identified 36Ar and 38Ar, yielding an isotopic ratio for 36Ar/38Ar of 5.4 ± 1.4, which is compatible with Solar System values: for Earth, this isotopic ratio is 5.3, while for the solar wind it is 5.5.
The relative abundance of argon to other gases was also investigated. For example, the abundance of argon relative to water vapour was determined to be between 0.1 x 10^–5 and 2.3 x 10^–5, the range of values measured being due to variable solar illumination, which influences the rate of water sublimation on different parts of the comet nucleus.
.
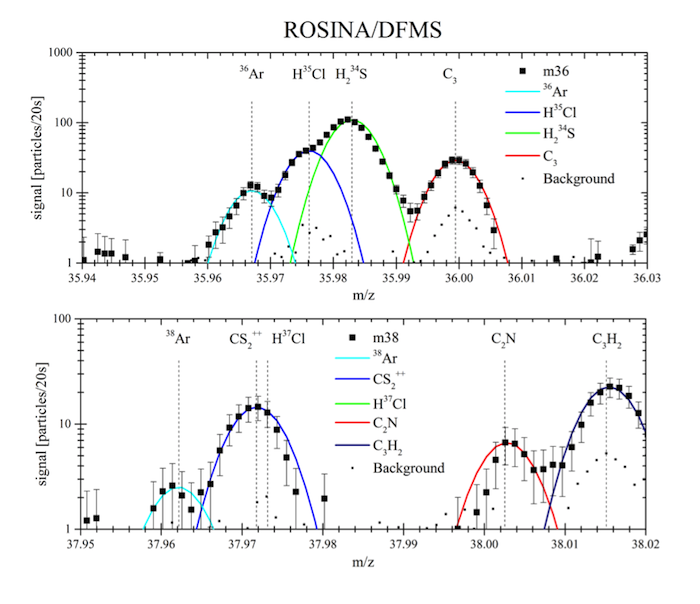
ROSINA-DFMS mass spectra identifying the two isotopes of 36Ar and 38Ar in October 2014, along with other gases. The extreme high mass-resolution of DFMS is a prerequisite for separating and identifying the two argon isotopes. The spacecraft background spectrum was obtained on 2 August 2014, before the comet signal became apparent. (m/z) = mass/charge. Data from Balsiger et al (2015).
.
“Even though the argon signal is very low overall, this unambiguous first in-situ detection of a noble gas at the comet demonstrates the impressive sensitivity of our instrument,” says Professor Kathrin Altwegg, principal investigator of the ROSINA instrument at the University of Bern.
“The argon-to-water ratio varied by more than a factor of 20. While the very volatile argon can escape under any conditions, water sublimation depends strongly on the amount of sunlight being received, and so with it the argon-to-water ratio,” explains Professor Hans Balsiger, also from the University of Bern, and lead author of the paper reporting the discovery.
“In contrast, the relative abundance of argon to molecular nitrogen is quite stable – explained by the fact that argon and nitrogen have similar high volatilities.”
Although the measured abundance of argon-to-water spans a wide range, it still has implications for the question of whether comets brought water to Earth. That is because the argon-to-water ratio at Earth is only 6.5 x 10^–8, several orders of magnitude lower than observed for 67P/C-G.
“The relatively high argon content of Comet 67P/C-G compared with Earth again argues against a cometary origin for terrestrial water, in an independent way to the similar finding indicated by the earlier ROSINA result on the deuterium-to-hydrogen ratio for 67P/C-G,” comments Hans.
The argon detection can also be used to learn about the conditions in which the comet formed.
“The argon we detected comes from inside the icy nucleus of the comet; the nature of that ice – how, when, and where it formed – determines how it captured and subsequently released the gases we are measuring” says Kathrin.
The two simplest forms of ice are crystalline and amorphous. These form at different temperatures and pressures, capturing and releasing gases in different ways. Argon, nitrogen, carbon monoxide, along with the heavier noble gases krypton and xenon are particularly useful for distinguishing between the various possibilities, because they remain in the same condition as when they were first incorporated into the comet.
Models can be used to predict how readily highly-volatile gases were incorporated into the icy grains that grew at low temperature in the protosolar nebula. These models show that the high abundance of argon at Comet 67P/C-G and the good correlation with nitrogen are both consistent with the comet forming in the cold outer reaches of the Solar System.
Almost a year has passed since these argon data were collected. Now that the comet has passed perihelion, its closest point to the Sun along its orbit, the density of the coma has increased greatly, implying that searches for even rarer gases should be possible.
However, the increased activity of 67P/C-G means that Rosetta cannot fly close to the comet without running into navigation issues, and therefore it is currently operating at distances greater than 350 km from the comet’s nucleus: this week, it has embarked on a trajectory taking it 1500 km from the comet in order to study the wider coma and plasma environment.
The ROSINA team are therefore eagerly waiting for Rosetta to return to closer distances as activity dies down in the coming months, in order to continue their investigation of the noble gases – including searching for krypton and xenon – to add further insights into the part played by comets in the delivery of these ingredients to Earth.
Quelle: ESA
-
Update: 29.09.2015
.
Churyumov-Gerasimenko: Rätsel um Form des "Quietsche-Enten-Kometen" gelöst
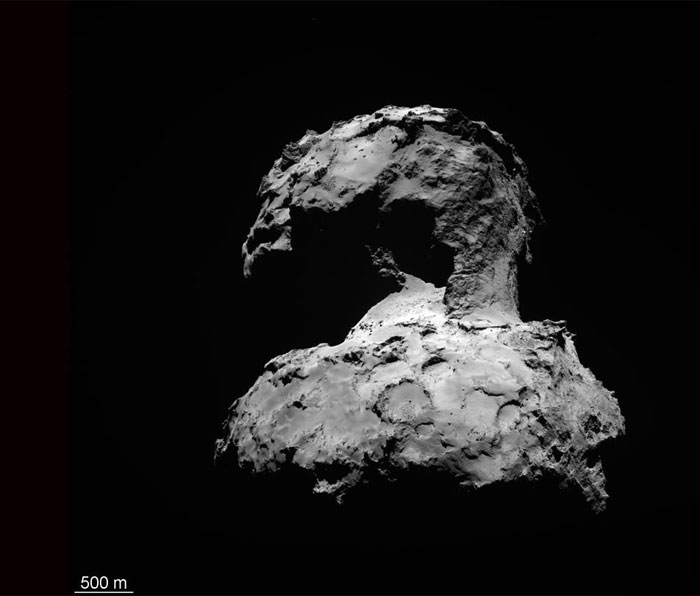
Von Gummientengestalt oder gar Quietsche-Entchen war in den Medien die Rede, als die überraschende Form von Komet 67P/Churyumov-Gerasimenko im Juli 2014 bekannt wurde. Auch die Wissenschaftler waren erstaunt über die außergewöhnliche Gestalt des Himmelskörpers, die die Raumsonde Rosetta offenbarte. "Sehr wahrscheinlich sind zwei Kometen im noch jungen Sonnensystem zusammengestoßen und bildeten den heute sichtbaren Doppelkörper", sagt Dr. Ekkehard Kührt, der die wissenschaftlichen Beteiligungen des Deutschen Zentrums für Luft- und Raumfahrt (DLR) an der Rosetta-Mission leitet. "Um die gemessene geringe Dichte und die gut erhaltenen Schichtstrukturen beider Kometenteile zu erklären, muss der Zusammenprall bei kleinen Geschwindigkeiten sehr sanft erfolgt sein. Diese Erkenntnis gibt wichtige Hinweise auf den physikalischen Zustand des frühen Sonnensystems vor 4,5 Milliarden Jahren", berichtet der Kometenforscher als Mitautor einer aktuellen Veröffentlichung im Fachjournal Nature.
Ursprünglich hatten die Wissenschaftler zwei Theorien im Visier: Sie vermuteten entweder eine Kollision zweier Körper oder eine besonders intensive Erosion an der Stelle, die sich schließlich zum Hals entwickelte. Die Analyse hochaufgelöster Bilder des Kometen von der OSIRIS-Kamera auf Rosetta, die zwischen dem 6. August 2014 und 17. März 2015 entstanden, brachte jetzt die Auflösung des Rätsels.
Ein Komet, zwei Körper
Zunächst hatten die Wissenschaftler auf den Bildern über hundert terrassenförmige Strukturen auf der Kometenoberfläche und parallel verlaufende Schichten ausgemacht, die deutlich an exponierten Klippen, Wänden und Vertiefungen zu sehen waren. Mithilfe eines 3D-Kometenmodells konnten sie anschließend schlussfolgern, in welche Richtung und in welcher Tiefe die einzelnen Schichten verlaufen. Schnell wurde klar, dass die schichtartigen Strukturen auf beiden Kometenhälften zu finden sind, sich dort aber im Detail voneinander unterscheiden. Das führte zu der Einsicht, dass sich die Strukturen nicht auf einem Körper gemeinsam entwickelt haben. Schon frühere Missionen zu den Kometen Tempel-1 und Wild-2 hatten Hinweise auf den schichtartigen Aufbau der Himmelskörper - ähnlich einer Zwiebel - geliefert.
Urmaterie des Sonnensystems
"Kometen gelten als Zeitzeugen der Bildung unseres Planetensystems, da sie sich durch ihre Entstehung in dessen kalten äußeren Regionen und wegen ihrer geringen Größe gut erhalten haben", erläutert Dr. Ekkehard Kührt vom DLR-Institut für Planetenforschung in Berlin. "Unklar war, inwieweit gegenseitige Stöße zur Alterung beigetragen haben. Die Daten der Rosetta-Mission unterstreichen, dass Kometen auch in dieser Hinsicht nur moderat verändert wurden und tatsächlich sehr ursprüngliches Material darstellen." Zudem deutet der ähnliche Aufbau beider Teilkörper darauf hin, dass diese einst in ähnlicher Weise entstanden sind.
Seit August entfernt sich Rosetta gemeinsam mit dem Kometen wieder von der Sonne und wird mit seinen elf Experimenten noch ein weiteres Jahr - bis Ende September 2016 - wissenschaftliche Daten sammeln.
Quelle: DLR
4033 Views