5.10.2020
A team of astronomers using the ESO instrument GRAVITY has taken the first image of an exoplanet that had previously only been detected indirectly via the spectrum of its star. The result is the first set of measurements that allows astronomers to both determine an exoplanet’s intrinsic brightness and estimate its mass. For the planet, beta Pictoris c, the outcome is surprising: Even though it may have a similar mass as its sister planet beta Pictoris b, its brightness is lower by a factor 6. All in all, beta Pictoris c poses a challenge to the classical model of planet formation by disk instability, and may be brighter than expected from the rival model of core accretion.
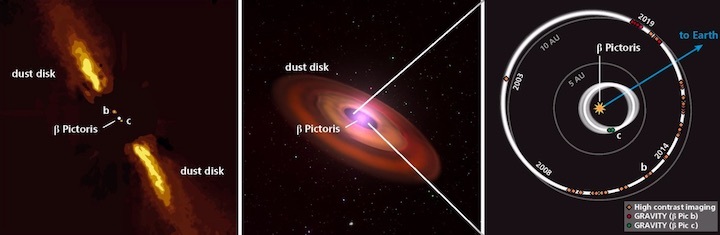
New observations using the GRAVITY instrument, which combines the light of all four 8-meter telescopes at ESO’s Paranal observatory in Chile, have resulted in the first direct observation of an exoplanet – a planet orbiting a star other than the Sun – that had previously been detected by the so-called radial velocity method. The radial velocity method observes the host star’s spectrum to find indirect clues to the presence of planets. As a bonus, it allows for an estimate of the masses of planets detected this way. A direct image of the planet, in turn, contains information about the planet’s intrinsic brightness. Note that intrinsic brightness means the light produced by the planet’s heat itself, due to the cooling of its interior – not the reflected or reemitted starlight of the planet. For young planets as in this case, the heat is a remnant of the planet formation process.
A planet catalog full of gaps
To appreciate what this new measurement means, consider what we know and do not know about the more than 4300 exoplanets discovered to date. Astronomers have a size (diameter) estimate for 70% of those planets, more than two-thirds. So far, so good. But there is a mass estimate for only one in five of those planets, and for only about 1% of planets there are direct images, allowing for measurements of a planet’s intrinsic brightness. Make a catalog of exoplanet properties, and most columns will be dominated by gaps.
The reason behind those gaps lies in the quirks of the different methods used to detect and study exoplanets. The transit method, where a planet is detected by the periodic minimal dimming of its host star’s light as the planet passes in front of the star, as seen by an observer on Earth, allows an estimate of a planet’s size – the larger the planet, the greater the amount of light it keeps from reaching us when it is between us and the star.
Take a precise spectrum of the star, and you can detect its planets with the radial velocity method: Saying that a planet “orbits its host star” is a simplification. In reality, both the planet and the star orbit a common center of mass. If the star, during that orbit, moves repeatedly a bit towards us and later away from us, that movement results in a periodic shift of clearly identifiable features (“spectral line”) in the star’s spectrum – the rainbow-like decomposition of its light. From those periodic shifts, the presence of an exoplanet can be inferred. All else being equal, a more massive planet will cause the star to move more widely, and the spectrum to shift more significantly, than a low mass planet. The stellar motion induced by the planet will likewise be faster if a planet orbits its star more closely. This motion allows for estimating the mass of planets discovered by that method. With additional information about the inclination of the planet’s orbital plane as seen by an observer on Earth (which can be provided either by the transit method or by direct observation), the data can be used for a proper measurement of the mass.
Last but not least, some few planets can be observed directly – by taking an image on which the planet appears as a small dot. This amounts to a measurement of the planet’s intrinsic brightness – as opposed, say, to the brightness due to reflected or reemitted starlight – which allows to infer the heat that the planet has retained from its formation.
Different methods, different strengths
So if those methods are complementary, why not use them all at the same time, obtain a planet’s size, intrinsic brightness and mass, and be done with it? The problem is that the different methods work differently well for different types of planet.
For instance, radial velocity methods will work well for planets that are closer to their host star, because the velocity change of the star during the orbit of the planet will be larger. Transit observations, where a planet passes in front of its star, also favor planets in close orbits, increasing the likelihood for the planet to pass directly in front of its star, from our perspective. As a result, astronomers now have both transit and radial velocity detections for around 800 planets, allowing them to measure both mass and size, and thus the planet’s density.
Direct images of planets, on the other hand, are very rare because it is very difficult to take a picture of a planet next to its bright host star star. Star’s are typically between a thousand and tens of billions times as bright as planets, and most planets are simply lost in their host star’s glare. Detection works best when the planet is very far away from its star, typically more than 10 times as far as the Earth from the Sun.
Of the 50 planets where there are images, only five have an orbital period of less than 20 years. The majority of those planets have periods of more than a hundred years. The longer the orbital period, the more difficult it is to capture the “wiggling” of the host star over a given period of time. Ideally, you want to follow the pattern for more than one whole period, to be sure of the periodicity.
In addition, direct imaging works best for younger stars, less than 100 million years old: for such young systems especially the gas giant planets still glow hot from formation and are easier to detect. Radial velocity measurements, on the other hand, work best for older stars. Younger stars typically exhibit stronger stellar activity, such as star spots or convection, which make the necessary spectral measurements more difficult
Difficulties of finding the right candidate
Astronomers looking for a planet where they could measure both mass and intrinsic brightness thus had to operate in a tight spot: How to find a planet distant enough for direct imaging, but not too distant for applying the radial velocity measurement, around a star that is young, but not too young?
This is where several things came together. In 2016, the GRAVITY instrument was commissioned at ESO’s Paranal Observatory. GRAVITY combines the light from the four 8-meter telescopes of the Very Large Telescope (VLT) using a method known as interferometry, allowing the instrument to distinguish details as small as if the observations were done by a telescope with a single mirror, 130 m in diameter. Interferometry is particularly well-suited to observing, and determining the distance between, two point-like sources, like a distant star and one of its planets.
Since 2019, the ExoGRAVITY project has used the instrument to find out the properties of known directly imaged exoplanets. For some planets, this has also included taking spectra, which might yield information about the composition of a planet’s atmosphere. Knowing the capabilities of their instrument, and its abilities to take images of planets that were close to their host stars, the ExoGRAVITY team had been on the look-out for a suitable candidate planet detected by the radial velocity method, that might yield a direct image.
The first double measurement of mass and brightness
In 2019, a group of astronomers led by Anne-Marie Lagrange at Grenoble Observatory announced the radial-velocity discovery of a new planet in the β Pic, or beta Pictoris, system. The detection was based on spectra taken over the preceding 16 years, with an estimated distance to its host star almost 3 times as far as the Earth is from the Sun. When they learned about the discovery, the ExoGRAVITY team suspected they might have found a suitable candidate for the combined measurement of mass and intrinsic brightness. In particular, the radial-velocity discoverers had already done part of the heavy lifting: The star β Pic is only 23 million years old – favourable for direct imaging, difficult for radial-velocity measurements. The astronomers analysing the spectra had to model stellar activity carefully in order to find the planet’s radial velocity signal.
At a distance of only 63 light-years, in the small Southern constellation “Painter” (lat. Pictor), the β Pic system is one of the closest young stellar systems. By the time of the new observations, it had already been discovered to have a debris disk produced by colliding small bodies, a giant planet designated beta Pictoris b, and evaporating comets.
This encouraged a group of astronomers led by Mathias Nowak of the University of Cambridge, which includes several researchers from the Max Planck Institute for Astronomy, to take aim at β Pic c. Making use of the unique capabilities of GRAVITY, and guided by updated position estimates derived from radial-velocity data by Lagrange and her colleagues published in a separate paper today, the astronomers managed to take images of the newly discovered planet for a total of three hours, which also provide a direct measurement of the planet’s intrinsic brightness.
"It is amazing, what level of detail and sensitivity we can achieve with GRAVITY", marvels Frank Eisenhauer, the lead scientist of the GRAVITY project at the Max Planck Institute for Extraterrestrial Physics. "We are just starting to explore stunning new worlds, from the supermassive black hole at the centre of our galaxy to planets outside the solar system."
This way, and for the first time, the astronomers obtained a combination of the mass and the intrinsic brightness for an exoplanet – the mass from the radial-velocity measurement plus some input from the direct image, and the brightness by direct measurement.
Homework for planet formation researchers
The brightness measurement came as something of a surprise. Compared to β Pictoris b, the planet β Pictoris c is rather faint. Both planets may have about the same mass (values ranging from 6 to 15 Jupiter masses for b, and 8 Jupiter masses for c), but b is six times brighter than c!
Generally speaking, astronomers expect giant planets, like β Pictoris b and c, to be brighter when they are more massive. But the precise relation between brightness and mass depends on how those planets have formed. Giant planets likely consist of a solid core, covered by enormous amounts of gas, and there are currently two competing classes of models for their birth: The “disk instability models” (which do not lead to such a solid core) posit that giant planets formed directly from the initial disk of gas and dust that surrounded the young star – gaseous atmosphere and all. The “core accretion models” posit that first, a planetary core forms out of solid material in the initial disk. That core later captures all the gas needed to make up a gas giant’s extensive atmosphere.
The two competing models make different predictions for the relationship between giant planets’ mass and brightness. Notably, the classical disk instability models lead to hotter, brighter planets for any given mass. This is where combined measurements of exoplanets’ mass and brightness can make a real difference, allowing astronomers to make deductions about modes of planetary birth.
At this time, only the mass estimate for β Pictoris c is reliable. It points to a hot origin of the planet, but somewhat cooler than expected from the disk instability models. The planet is also hotter than what the classical formation models for core accretion predict – but there are newer core accretion models that would allow for that kind of hotter evolution. Also, the current orbit of β Pictoris c orbit is far too close to its star for planet formation via disk instability, so that mode of birth would require the planet to have migrated inwards significantly after its formation – another complication. Taking all this information together, the authors favour the core accretion way for forming β Pictoris c.
Paul Mollière, a postdoctoral researcher at the Max Planck Institute for Astronomy and co-author of the article describing the new results, says: “We used GRAVITY before to obtain spectra of other directly imaged exoplanets, which themselves already gave useful hints regarding their formation process. This brightness measurement of beta Pictoris c, combined with its mass, is a particularly important step to constraining our planet formation models.”
Future directions
In following up on their pioneering measurements, the astronomers will next direct their attention to β Pictoris b – when future observations, following both the planet’s position and the star’s radial velocity over a longer time, lead to a reliable mass estimate for that planet, the astronomers will have an additional data point to test planet formation models. All in all, planets allowing for measurements of both mass and intrinsic brightness are expected to remain very rare indeed, although the ExoGRAVITY team has at least one additional candidate in view, where they are aiming for this kind of measurement.
In parallel, the team is working on an additional line towards more information: GRAVITY allows for the determination of spectra for an exoplanet, splitting the planet’s light into its different wavelengths. Such spectra contain information about the composition of exoplanet atmospheres. The GRAVITY spectra for β Pictoris c, which have already been obtained, are very special indeed – so far, the only known case where we can obtain spectra for a planet in a Jupiter-like orbit within its system. Other directly imaged planets are much more distant from their host star than β Pictoris c, while spectra taken using the transit method are from planets much closer to their star.
Understanding that composition, in turn, represents a key step towards a more fundamental goal: honing our capabilities of constraining atmospheric compositions may ultimately allow us to detect chemical traces of life within an exoplanet atmosphere, providing evidence for life outside the solar system.
Quelle: MAX-PLANCK-GESELLSCHAFT, MÜNCHEN