29.07.2023
A vast telescope buried beneath Antarctica has captured high-energy neutrinos from the Galactic Centre, ushering in a new era for observing the Universe.
An artist’s concept of what the plane of the Milky Way would look like if viewed using neutrinos (blue).Credit: IceCube Collaboration/NSF (Lily Le & Shawn Johnson)/ESO (S. Brunier)
In June, researchers released what might be the most unusual portrait of the Milky Way yet. For the first time, astronomers have charted our Galaxy using something other than light rays — a finding that heralds a new way of studying what goes on in the busy centre of the Milky Way.
The data came from the IceCube observatory at the South Pole, which detects neutrinos, the lightest, most pervasive of the Universe’s elementary particles. The observatory is a vast array of 5,160 sensors, buried more than 1.5 kilometres below the surface of the ice sheet. Over the course of ten years, the array detected neutrinos one at a time and measured the direction they came from, which allowed researchers to chart their origin to the centre of the Milky Way.
The first neutrino map of our Galaxy is a milestone for the nascent field of neutrino astronomy, say researchers. And it’s just the start. Construction is under way on several neutrino-detecting arrays, at sites from the Mediterranean Sea to Siberia.
Neutrinos are known to arise through myriad subatomic processes: from nuclear fusion in the Sun’s core and radioactivity in Earth’s rocks to high-energy collisions of interstellar particles with the atmosphere. In the past decade, the IceCube Neutrino Observatory has looked deeper into space, revealing neutrinos with record-high energies, and has pinpointed their origins to distant cosmic sources.
In fact, it has been easier for astronomers to spot neutrinos from the far reaches of the Universe than those from the Milky Way. “We now have more information on the extragalactic sources than the Galactic ones,” says IceCube spokesperson Francis Halzen, a physicist at the University of Wisconsin–Madison. “Which is amazing.”
IceCube and other observatories are turning neutrinos into a tool for peering into otherwise inaccessible places, such as the dense maelstroms of matter swirling around supermassive black holes at the centres of galaxies, where extreme energies could reveal new physical phenomena. And the approach could ultimately help to unveil the source of cosmic rays — protons or heavier atomic nuclei that move at nearly the speed of light.
High-energy focus
IceCube was completed in 2010, built to capture neutrinos from deep space. Its goal is to study a broad range of cosmic phenomena that might produce neutrinos of high energies — thousands of times more energetic than those that form during nuclear fusion in stars or as a result of radioactivity, say.
Mapping the Galaxy using high-energy neutrinos was always a top priority, says Halzen, in part because it could shed light on a long-standing mystery. Interstellar space is filled with high-energy cosmic rays, and researchers don’t know for sure where they come from. But one thing is clear: when they collide with other matter, such particles must produce an abundance of high-energy neutrinos — the ones that IceCube was designed to see, each packing energies of 500 gigaelectronvolts (GeV) or more.
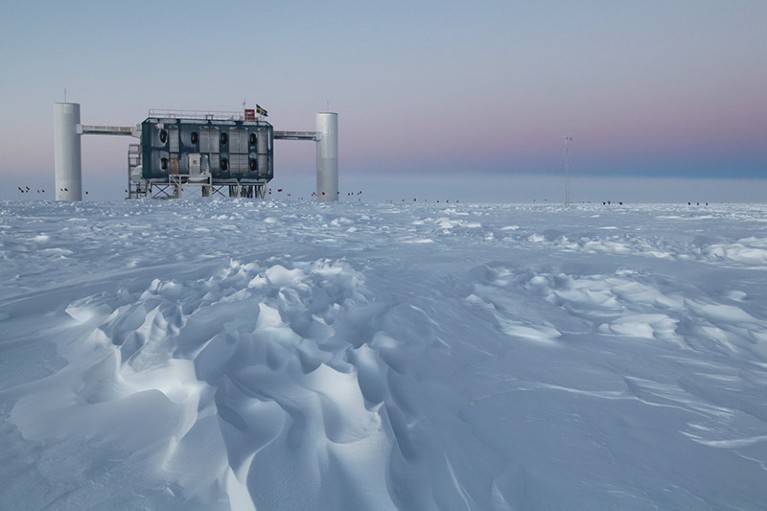
The IceCube Neutrino Observatory at the South Pole.Credit: Sven Lidstrom, IceCube/NSF
This idea is consistent with maps that show much of the Galaxy as having a diffuse glow of γ-rays, which are another expected by-product of cosmic rays. So, IceCube researchers expected to see intense neutrino emissions from the same regions of the sky. “We know that these neutrinos must be produced within the Galaxy,” says Teresa Montaruli, an IceCube physicist at the University of Geneva in Switzerland.
But tracing neutrinos back to the Milky Way posed multiple challenges. The first is that the majority of the neutrinos the observatory sees are created in the atmosphere, at a rate of roughly one every 5 minutes, explains Halzen — much greater than the rate expected from deep space. That means researchers had to collect many years’ worth of data before a signal would start to emerge.
A second challenge is geography. IceCube gets its cleanest neutrino detections from underneath. That’s because neutrinos are the only known particles that can travel through the entire planet unimpeded and reach the South Pole ice from beneath. This means that signals from points below the horizon are easier to distinguish from the cacophony of particles that hit the detector from above, which mostly come from the massive cousins of electrons called muons. But to see the Milky Way, IceCube did not have the luxury of using Earth’s mass as a shield. Most of the Galaxy’s mass is concentrated in one strip of the southern sky around the constellation of Sagittarius — permanently above the horizon as seen from the South Pole.
So the team had a massive sorting job to find the high-energy Galactic neutrinos. The researchers analysed 59,592 detections between May 2011 and May 2021, in the energy range of 500 GeV to several petaelectronvolts. They estimated that only 7% of those were neutrinos originating from deep space. They then used machine-learning techniques to show that, whereas most of the events had points of origin scattered across the entire sky, a small number were concentrated in regions of the Milky Way that also have high γ-ray emissions, which indicates that some must have been neutrinos from the Galaxy (see ‘Different views of the Galactic Centre’). The team reported the results in the 30 June issue of Science1.
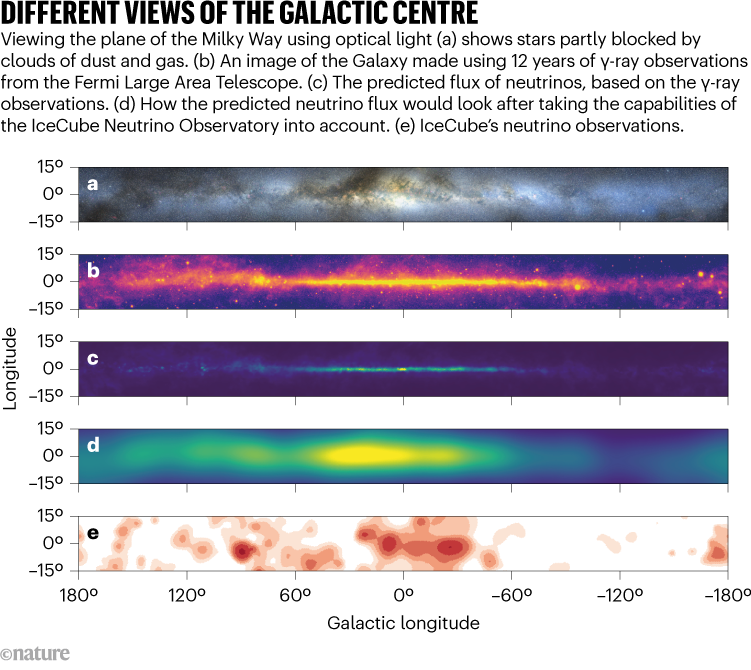
Source: Ref. 1; IceCube/NASA/DOE/Fermi LAT Collaboration/Axel Mellinger, CMU
The study did not reveal any concentrated sources, suggesting that the neutrinos were created in interstellar space. But Halzen hopes that further data — and an upcoming upgrade that will make the observatory sensitive to neutrinos of lower energies — will reveal some. Finding point sources in the Milky Way could begin to solve the origin of cosmic rays — and perhaps even reveal unsuspected neutrino factories that have nothing to do with cosmic rays at all.
Starry messengers
Researchers have high hopes for using neutrinos as discovery tools, because these electrically neutral particles don’t get rerouted by the Galaxy’s magnetic fields, which tend to scramble the motions of charged particles. “Unlike cosmic rays, neutrinos travel in straight trajectories and hence they are optimal messengers to unveil the acceleration sites” of cosmic rays, says Silvia Mollerach, an astrophysicist at the Balseiro Institute in San Carlos de Bariloche, Argentina.
So far, attempts to locate the point sources of cosmic rays have been unsuccessful. IceCube itself seems to have ruled out one phenomenon that astrophysicists used to see as promising: the extremely energetic supernova explosions called γ-ray bursts. Year after year, the observatory has failed to link any neutrinos to these events. The most stringent limits yet on how many high-energy neutrinos emerge from γ-ray bursts came from the most powerful burst seen so far, observed in 2022.
Although the bursts and other stellar explosions release stupendous amounts of energy in a short time, they might not accelerate individual protons to the near-light speed required to make cosmic rays and neutrinos, Halzen says.
The long-term aftermath of a supernova could be a different matter, however. Over centuries, the expanding shock waves from such an explosion could act as pinball machines, accelerating protons to higher energies. “Each time the proton crosses the shock wave, it gains a bit of energy,” says Montaruli.
IceCube has been much more successful at locating point sources outside the Galaxy, specifically those in active galactic nuclei (AGN)— supermassive black holes that emit bright radiation as they gobble large amounts of matter from their surroundings.
In 2018, the collaboration linked a single, extremely energetic neutrino to a blazar event — a flaring from an AGN that temporarily makes it even brighter2. And last year, the team announced that it had collected around 80 neutrinos coming from the AGN of the Squid galaxy, also known as NGC 10683.
Surprisingly, however, the heart of the Squid is not bright in γ-rays. “NGC 1068 seems to be a neutrino source, but the photons that should be produced in the same interactions don’t come through,” says Elisa Resconi, an astroparticle physicist at the Technical University of Munich in Germany. This means its central black hole must be surrounded by a layer of dust thick enough to block γ-rays. This makes neutrinos all the more valuable, because they are the only medium available to understand the physics around the giant black hole, Resconi says. At a distance of just 14.4 megaparsecs from Earth, the Squid is one of the nearest and best-studied AGNs.
Resconi is spokesperson for the Pacific Ocean Neutrino Experiment, a proposed neutrino observatory in the deep ocean off Vancouver Island, Canada, that would have an even larger volume than IceCube — more than 2 cubic kilometres. Another large neutrino observatory, called the Cubic Kilometre Neutrino Telescope, is being built at three separate locations in the Mediterranean Sea. And Russia is constructing a cubic-kilometre neutrino observatory in Lake Baikal in Siberia. Together, these observatories would form a network that could collect unprecedented numbers of neutrinos from across the sky.
The findings from the Squid galaxy are the kind of discovery that make astronomers especially excited about neutrino astronomy. The extreme environments surrounding black holes offer natural laboratories for testing the limits of the known laws of physics, and open up the possibility of finding new ones, says Resconi. “For a scientist, it doesn’t get any better than this.”
Quelle: nature